In a groundbreaking study, an international team of scientists has achieved the first-ever detection of a mid-infrared (Mid-IR) flare from Sagittarius A* (Sgr A*), the supermassive black hole located at the center of the Milky Way. This discovery, made possible by the Mid-Infrared Instrument (MIRI) aboard the James Webb Space Telescope (JWST), provides a new and important insight into the nature of the flares emitted by Sgr A*. Simultaneous radio observations also revealed a counterpart flare in the radio wavelengths, which lagged behind the mid-IR flare, offering further clues about the mechanisms at work. The findings have been published on the arXiv preprint server.
Sagittarius A* and Its Flares: A Longstanding Mystery
Sgr A*, with a mass of approximately 4 million times that of our Sun, has been a subject of intense study for several decades. Since the early 1990s, astronomers have observed this supermassive black hole as it regularly exhibits flares—bursts of high-energy radiation emitted from its accretion disk, the region surrounding the black hole where matter is being drawn in by gravity. These flares have been detected in various wavelengths, from radio waves to X-rays, providing a broad view of Sgr A*’s behavior across the electromagnetic spectrum.
Despite decades of successful observations and even capturing the first-ever image of Sgr A*’s shadow by the Event Horizon Telescope in 2022, one key piece of the puzzle remained elusive: mid-infrared observations. Until now, no team had managed to detect variability in Sgr A* at these wavelengths, creating a gap in our understanding of the mechanisms that drive the flares and how they evolve. This gap in data has left scientists with questions about the true nature of the flares and the completeness of the theoretical models explaining them.
The Role of Mid-Infrared Observations
Infrared light, which has longer wavelengths than visible light but shorter wavelengths than radio waves, is an important tool for astronomers. Mid-infrared (Mid-IR) light, in particular, sits between the submillimeter and near-infrared (NIR) portions of the spectrum. It allows scientists to study regions of space where objects are often obscured by dust, which can block the view in other wavelengths. This makes Mid-IR a crucial tool for observing flares from supermassive black holes like Sgr A*, which are often shrouded in dust.
Before this study, scientists had observed flares from Sgr A* in other wavelengths, including radio and near-infrared, but had not been able to detect variability in the mid-IR range. The lack of this data left a significant gap in understanding the flaring process. The recent discovery by the team using the MIRI instrument on JWST fills this gap, offering a fresh perspective on how Sgr A* emits energy.
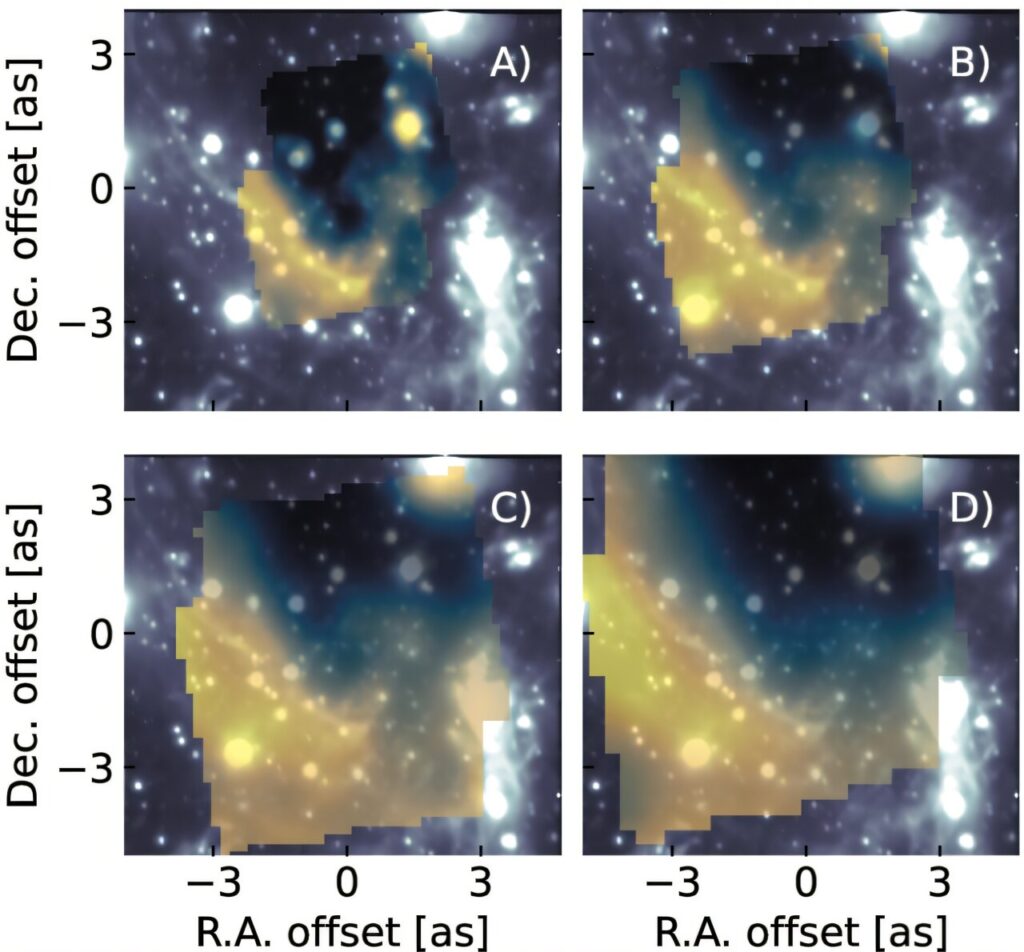
Understanding the Flares: Theories and Models
Although scientists have observed flares from Sgr A* for many years, the exact cause of these flares remains uncertain. Researchers rely on theoretical models and computer simulations to help explain the mechanisms behind the flaring activity. One widely accepted model suggests that the flares are the result of magnetic reconnection in the turbulent accretion disk surrounding the supermassive black hole.
In this model, the magnetic field lines within the accretion disk become tangled due to the extreme environment around the black hole. When two magnetic field lines come close to each other, they can reconnect, releasing vast amounts of energy. This energy release accelerates electrons to near the speed of light, and these energized electrons move along the magnetic field lines. As they travel at such high speeds, they emit high-energy radiation, a process known as synchrotron emission, which powers the flares observed from Sgr A*.
The recent observations of a mid-IR flare from Sgr A* are consistent with this model. The data suggests that the flare is indeed the result of synchrotron emission, powered by cooling electrons that lose energy as they move through the magnetic field. The new detection of mid-IR variability supports the existing theoretical models, providing further evidence for the role of synchrotron radiation in these dramatic outbursts.
The Importance of Simultaneous Multi-Wavelength Observations
A key aspect of this study is the use of simultaneous observations across multiple wavelengths. While the mid-IR flare was detected by the James Webb Space Telescope, the team also conducted radio observations with the Submillimeter Array (SMA), X-ray observations with the Nuclear Spectroscopic Telescope Array (NuSTAR), and the Chandra X-ray Observatory. These simultaneous observations provided a more complete picture of the flare and its behavior across different parts of the electromagnetic spectrum.
One of the most interesting findings from the multi-wavelength observations was the lag observed between the mid-IR flare and its radio counterpart. The radio flare was detected by the SMA about 10 minutes after the mid-IR flare. This time lag suggests a complex relationship between the processes that generate flares at different wavelengths, further supporting the idea that synchrotron emission is at play. The lag may indicate that the high-energy electrons responsible for the flare emit radiation at different wavelengths as they cool and lose energy.
While the X-ray observations did not detect any flare during this event, the team theorizes that this may be because the flare did not accelerate electrons to the extremely high energies required to produce X-rays. However, the detection of the radio flare in conjunction with the mid-IR flare helps to confirm that the two wavelengths are related and arise from similar processes.
Filling the Gap in Our Understanding
The successful detection of mid-IR flares from Sgr A* is a significant step forward in our understanding of the processes occurring near supermassive black holes. The results not only confirm the role of synchrotron radiation in the generation of flares but also offer new insights into the dynamics of magnetic reconnection and the turbulence in the accretion disk.
“This first-ever mid-IR detection, and the variability seen with the SMA, has not only filled a gap in our understanding of what has caused the flare in Sgr A*, but has also opened a new line of important inquiry,” said Sebastiano von Fellenberg, a postdoctoral researcher at the Max Planck Institute for Radio Astronomy (MPIfR) and the lead author of the paper.
The results underscore the importance of multi-wavelength observations in studying supermassive black holes. By observing Sgr A* across a range of wavelengths, scientists can gain a clearer and more comprehensive picture of the physical processes at work in the black hole’s accretion disk. This approach is not only crucial for understanding Sgr A* but will also be important for studying other supermassive black holes, such as M87*, which are known to exhibit similar flare activity.
A New Era of Observation with the James Webb Space Telescope
The success of this study highlights the extraordinary capabilities of the James Webb Space Telescope. The telescope’s advanced Mid-Infrared Instrument (MIRI) has enabled scientists to probe the region around Sgr A* with unprecedented detail and sensitivity. The quality of the mid-infrared data collected by JWST is a testament to the technical capabilities of the telescope, which continues to push the boundaries of what we can observe in the universe.
“Working on reducing and calibrating the data from James Webb—which is presently one of the best telescopes we have—was a dream come true for me,” said Tamojeet Roychowdhury, a student from the Indian Institute of Technology in Bombay and a contributor to the study. “We are building an increasingly detailed picture of the processes that take place in the immediate vicinity of a supermassive black hole.”
Reference: Sebastiano D. von Fellenberg et al, First mid-infrared detection and modeling of a flare from Sgr A*, arXiv (2025). DOI: 10.48550/arxiv.2501.07415
Behind every word on this website is a team pouring heart and soul into bringing you real, unbiased science—without the backing of big corporations, without financial support.
When you share, you’re doing more than spreading knowledge.
You’re standing for truth in a world full of noise. You’re empowering discovery. You’re lifting up independent voices that refuse to be silenced.
If this story touched you, don’t keep it to yourself.
Share it. Because the truth matters. Because progress matters. Because together, we can make a difference.
Your share is more than just a click—it’s a way to help us keep going.