Spintronic devices have garnered significant attention in recent years due to their ability to harness the spin of electrons, rather than their charge, to process information. One such device, the Spin Hall Nano-Oscillator (SHNO), has shown tremendous potential for applications in neuromorphic computing, telecommunications, and optimization problems like those tackled by Ising machines. These nanoscale devices generate high-frequency microwave signals from direct current through nonlinear magnetization oscillations known as spin waves. SHNOs are self-sustained and do not require an external periodic force, making them particularly efficient for certain applications.
A new study, led by researchers at the University of Gothenburg and Tohoku University, has advanced the understanding of SHNOs by demonstrating the coupling between SHNOs through propagating spin waves. This breakthrough could lead to significant advancements in the design of more synchronized, scalable spintronic devices, offering new opportunities for optimized computation and efficient signal transmission.
What are Spin Hall Nano-Oscillators (SHNOs)?
At their core, SHNOs are spintronic devices that convert direct current (DC) into high-frequency microwave signals through a process known as spin-wave auto-oscillations. These oscillations are a type of nonlinear magnetization oscillation in which the spins of electrons precess at a high frequency, generating electromagnetic waves. These oscillations are not externally driven but are instead self-sustaining, meaning the devices can generate microwave signals without the need for a periodic force.
SHNOs typically operate on a nanoscale and are often composed of layers of magnetic materials such as W/CoFeB/MgO, where W represents Tungsten, CoFeB is a cobalt iron boron alloy, and MgO is magnesium oxide. When a current flows through these materials, it can induce a spin Hall effect, which in turn excites the magnetization of the material, generating spin waves. These spin waves are essential to the functionality of SHNOs, as they mediate the exchange of information between multiple devices, facilitating synchronization and coupling.
The Role of Propagating Spin Waves in SHNOs
While SHNOs have been studied for years, one of the major challenges in the field has been how to control the synchronization and coupling between multiple SHNOs. In previous studies, SHNOs could synchronize with each other over short distances but were limited by the nearest-neighbor coupling, where only adjacent SHNOs could be coupled effectively. This limitation made scaling up systems involving multiple SHNOs for practical applications such as neuromorphic computing or optimization tasks difficult.
However, theoretical and simulation studies indicated that propagating spin waves, which travel across the material instead of being confined to the auto-oscillation region, could enable long-range coupling between SHNOs. This type of propagating coupling would make it possible to control the synchronization between SHNOs that are further apart, opening new doors for scalable, large-scale systems of SHNOs.
The study conducted by the teams at the University of Gothenburg and Tohoku University experimentally demonstrated spin-wave mediated SHNO-to-SHNO coupling, a novel mechanism that was previously theorized but not fully realized. This spin-wave coupling provides a means of dynamically adjusting the timing and phase of oscillations between SHNOs, which is a significant step forward for applications in neuromorphic computing and optimization hardware.
Experimental Breakthrough: Demonstrating Propagating Spin Waves in SHNOs
The researchers’ journey began with a crucial prior achievement: the realization of propagating spin waves in SHNOs. In earlier work, the team successfully demonstrated that spin waves could travel through optimized thin-film samples of materials like W/CoFeB/MgO, showing that spin waves were not simply confined to the region where oscillations were initiated but could propagate across the device.
Building on this foundational research, the team sought to use propagating spin waves to mediate the coupling between two SHNOs. Their approach involved two SHNOs that were fabricated using standard nano-fabrication processes. The researchers fine-tuned the magnetic anisotropy of the SHNOs and adjusted the distance between them to achieve the desired mutual synchronization. This synchronization was governed by the phase and frequency of the spin waves, which allowed the oscillators to communicate and transfer information.
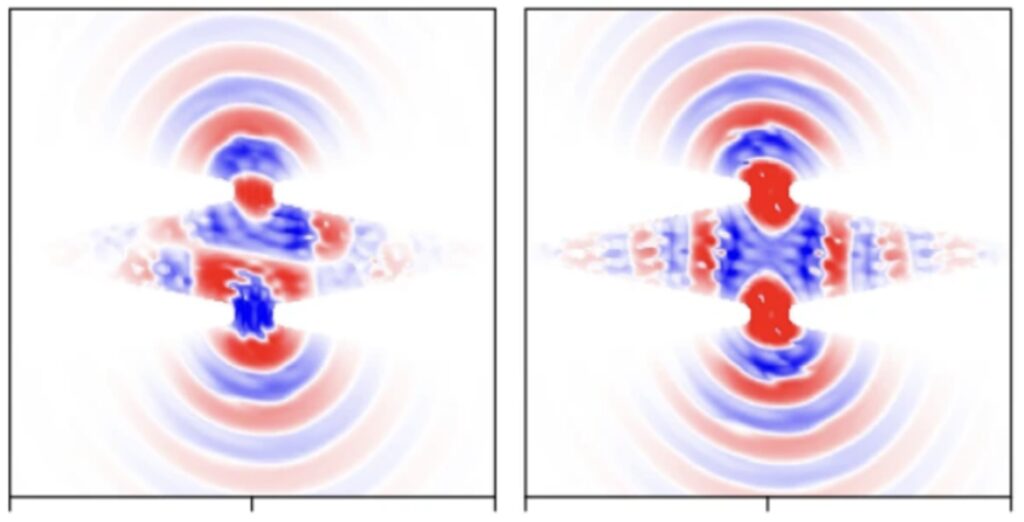
Using high-frequency spectrum analyzers, the researchers first observed phase-tuned mutual synchronization in the electrical measurements, where the power spectral density showed clear evidence of synchronized oscillations between the two devices. This was an initial indicator that the SHNOs were interacting in a way that allowed them to synchronize their oscillations.
Phase-Resolved Microscopy and Simulations
To confirm their findings, the researchers turned to phase-resolved Brillouin light scattering (μ-BLS) microscopy. This advanced technique allowed them to directly measure and visualize the phase of each oscillator. The μ-BLS measurements provided the visual confirmation needed to validate the presence of propagating spin waves and their role in the synchronization process.
In addition to experimental measurements, the team ran micromagnetic simulations to further confirm their hypothesis and investigate the underlying mechanisms of the spin-wave mediated coupling. These simulations, conducted by graduate student Victor González, supported the experimental data and provided a detailed view of how the spin waves could transfer phase information from one SHNO to another, reinforcing the concept of long-range coupling between SHNOs.
Applications and Future Directions
The ability to dynamically control the coupling between SHNOs using propagating spin waves opens up new possibilities for the design of complex spintronic systems. In particular, the researchers noted the potential for these systems to be used in Ising machines, which are a type of combinatorial optimization hardware. Ising machines solve complex optimization problems by simulating the behavior of spins in a magnetic system, and SHNOs could be used to accelerate these processes in a scalable, energy-efficient way.
The researchers also highlighted the importance of further scaling up the system. Voltage control over the coupling between SHNOs could provide an energy-efficient means of on-demand control over the synchronization, making SHNO devices even more practical for real-world applications. By incorporating large numbers of SHNOs into chains or two-dimensional arrays, the team envisions a future where these systems could solve optimization problems much more efficiently than current methods allow.
“Our next studies will focus on incorporating a larger number of SHNOs and utilizing voltage gating to provide energy-efficient, local control of coupling between the oscillators,” said Akash Kumar, the first author of the study. “These advancements will make these devices functional for real-world applications in fields like neuromorphic computing, optimization, and signal processing.”
Conclusion
The experimental demonstration of spin-wave mediated SHNO-to-SHNO coupling represents a significant advancement in the field of spintronics. By using propagating spin waves to synchronize oscillators over long distances, researchers have overcome previous limitations in SHNO coupling and opened the door to more complex, scalable systems of spintronic devices. This research has the potential to impact a wide range of applications, including neuromorphic computing, optimization hardware, and microwave signal generation.
As the technology continues to evolve, the ability to dynamically control SHNOs using voltage and spin-wave coupling will undoubtedly play a key role in the development of next-generation devices. With continued progress in these areas, SHNOs could be poised to revolutionize fields such as combinatorial optimization, telecommunications, and artificial intelligence, bringing us closer to more efficient, powerful computing systems.
Reference: Akash Kumar et al, Spin-wave-mediated mutual synchronization and phase tuning in spin Hall nano-oscillators, Nature Physics (2025). DOI: 10.1038/s41567-024-02728-1.