In a significant stride toward advancing quantum computing, a team of applied physicists at the Harvard John A. Paulson School of Engineering and Applied Sciences (SEAS) has developed an innovative photon router capable of bridging the gap between optical and microwave photons. This breakthrough, described in a recent Nature Physics paper, holds immense potential for the future of quantum networks, creating robust optical interfaces for noise-sensitive microwave quantum computers and offering new pathways for scalable, modular quantum computing.
The Quest for Modular Quantum Networks
The dream of a future where quantum computers collaborate across large, distributed networks is inching closer to reality, thanks in part to this pioneering development. Imagine a world where quantum computing clusters, far-flung across cities or even continents, communicate seamlessly with one another through quantum links built on the backbone of existing telecommunications infrastructure. This vision may seem far off, but the work being done at Harvard’s SEAS is helping lay the groundwork for such systems.
Currently, the global fiber-optic networks, which span millions of miles of optical fiber, serve as the rapid and reliable communication highways that carry information as pulses of light. In this vast network, photons act as the primary messengers, rapidly transmitting data in the blink of an eye. In parallel, the rise of quantum computing, with its ability to perform calculations far beyond the reach of classical systems, requires a new form of communication that can handle quantum bits—or qubits. These qubits operate in an entirely different realm of physics, governed by the strange principles of quantum mechanics. Connecting them across vast distances without introducing interference or loss has long been a major challenge. But the breakthrough made by Marko Lončar, the Tiantsai Lin Professor of Electrical Engineering and Applied Physics at SEAS, and his team could be the answer.
Introducing the Microwave-Optical Quantum Transducer
The team’s breakthrough is the creation of a microwave-optical quantum transducer, a device that can serve as a kind of router for photons. This new technology acts as a bridge between the microwave and optical realms of quantum information, opening the door to more effective quantum communication. For decades, scientists have known that there’s a significant energy gap between microwave and optical photons, with the latter being much higher in frequency. As such, controlling superconducting qubits—those tiny circuits that represent quantum bits in microwave quantum computers—using only optical signals has always been an enormous challenge.
Now, with this new transducer, scientists can control microwave qubits using light, potentially transforming how quantum networks operate. The transducer acts as a mediator, enabling the use of optical signals generated miles away to control superconducting qubits in a quantum processor. It’s the first device of its kind to demonstrate this capability, making it an essential step toward more scalable and reliable quantum computing systems.
Graduate student Hana Warner, the first author of the paper, explained the significance of this advancement: “The realization of these systems is still a ways out, but in order to get there, we need to figure out practical ways to scale and interface with the different components. Optical photons are one of the best ways you can do that, because they’re very good carriers of information, with low loss, and high bandwidth.”
Superconducting Qubits: The Power and Challenges
Superconducting qubits have emerged as one of the most promising platforms for quantum computing due to their scalability and compatibility with existing manufacturing techniques. These qubits, made from superconducting materials, represent the quantum version of classical bits—essentially the smallest unit of quantum information. They can exist in multiple states simultaneously, a phenomenon known as superposition, which is the core of quantum computation.
One of the major advantages of superconducting qubits is their ability to maintain coherence—keeping their quantum properties intact long enough to perform meaningful calculations. However, there’s a significant hurdle: superconducting qubits need to be kept at extremely low temperatures to operate effectively. This requires large, complex cooling systems known as dilution refrigerators. As the number of qubits needed for large-scale quantum computers grows into the millions, relying solely on microwave-frequency signals for control becomes increasingly difficult.
The solution to this challenge lies in harnessing the power of optical photons to serve as a more efficient, scalable interface. Optical photons, unlike their microwave counterparts, can travel long distances with low energy loss and high bandwidth, making them perfect candidates for linking qubits in remote quantum computing clusters. However, until now, there was no clear method to link optical photons with the superconducting qubits that form the backbone of these quantum processors. The new microwave-optical quantum transducer bridges this gap, offering a practical solution for the challenges of scaling quantum systems.
How the Transducer Works: The Heart of the Innovation
The device designed by the Harvard team is a 2-millimeter optical chip, roughly the size of a paperclip, attached to a 2-centimeter chip. It functions by connecting a microwave resonator with two optical resonators. The material at the core of this breakthrough is lithium niobate, a crystal that enables efficient energy transfer between microwave and optical photons. This transducer allows for a back-and-forth exchange of energy between these two types of photons, opening up the possibility for seamless communication between different quantum computing nodes.
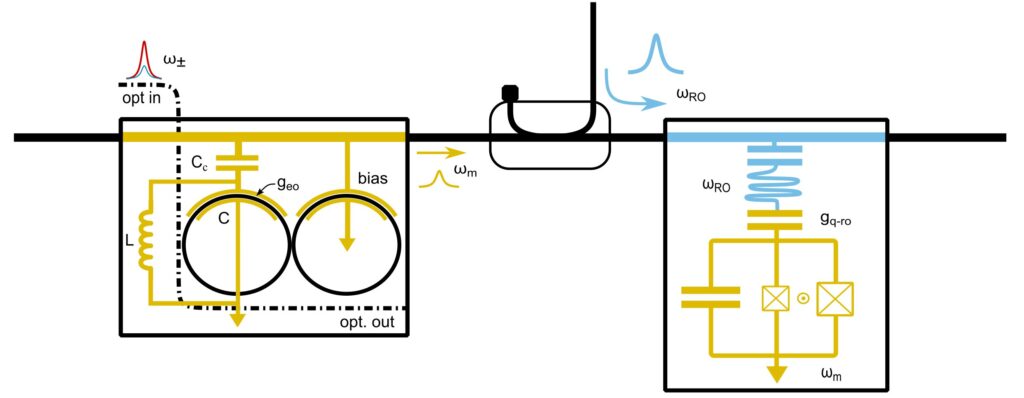
By linking microwave and optical resonators, the transducer eliminates the need for bulky microwave cables typically used to control the states of qubits. This is a significant step toward building more efficient quantum computers. The Harvard team envisions that their transducer could not only be used to control qubits but also for reading out qubit states or converting delicate quantum information into more stable packets of light. This could allow quantum information to be shared reliably across quantum networks, providing a vital link between superconducting quantum processors.
Collaborating for Success: A Multidisciplinary Effort
The success of the transducer hinges on the combined expertise of a multidisciplinary team. In addition to the Harvard SEAS researchers, the team collaborated with Rigetti Computing, which provided the aluminum-on-silicon superconducting qubit platforms that were crucial for testing the device. The researchers also worked with scientists from the University of Chicago and Massachusetts Institute of Technology (MIT), further expanding the breadth of knowledge and resources applied to this cutting-edge project.
The fabrication of the chips was conducted at Harvard’s Center for Nanoscale Systems, part of the National Nanotechnology Coordinated Infrastructure Network. This state-of-the-art facility provided the necessary tools to create the precise nanoscale components required for the transducer.
The Road Ahead: Quantum Networks on the Horizon
While the transducer represents a monumental step in the quest to build scalable quantum computing networks, it is only one part of a much larger puzzle. Marko Lončar, the lead researcher on the project, pointed out that the next step for the transducer is to enable the reliable generation and distribution of quantum entanglement between microwave qubits using light. Quantum entanglement—the phenomenon where particles become correlated in such a way that the state of one instantaneously affects the state of another—is a crucial resource for quantum computing. Its manipulation and distribution are key to realizing large-scale quantum networks.
The long-term goal of this research is to build a global quantum internet, where quantum computers can communicate and collaborate seamlessly over vast distances. By developing interfaces that can convert fragile quantum information into stable optical signals, researchers are paving the way for more reliable, efficient, and scalable quantum systems.
Conclusion: A New Era for Quantum Computing
The development of the microwave-optical quantum transducer by the Harvard SEAS team marks a critical milestone in the journey toward scalable, distributed quantum computing. By leveraging the properties of optical photons, researchers have unlocked the potential to connect quantum processors over long distances, bypassing some of the most significant obstacles to building practical quantum networks. As researchers continue to refine this technology and explore new ways to integrate it into larger quantum systems, the future of modular, interconnected quantum computing looks brighter than ever.
Reference: Coherent control of a superconducting qubit using light, Nature Physics (2025). DOI: 10.1038/s41567-025-02812-0