In the invisible world of molecules, some structures are less like rigid statues and more like acrobatic performers—twisting, turning, interlocking, and responding to subtle nudges from their environment. Among the most dazzling of these molecular contortionists are catenanes, a class of organic compounds whose key feature isn’t just how they’re built, but how they’re mechanically entwined. Like a pair of Olympic gymnasts permanently linked at the wrists, these molecules are composed of interlocked rings that can’t be pulled apart unless their covalent bonds are broken—a task requiring deliberate chemical intervention.
These ring-shaped structures don’t merely coexist; they are mechanically interlocked, meaning their very existence as a single entity depends on their entanglement. But now, researchers are delving deeper than ever before—not just into how these catenanes hold together, but how their dynamic structure can generate chirality—a mirror-image asymmetry that has profound implications across chemistry and biology.
Chirality: More Than a Twist of Fate
In traditional chemistry, chirality is often about shape. Think of your hands—they are mirror images of each other, yet you can’t superimpose one perfectly over the other. This kind of non-superimposable asymmetry also appears in molecules and is crucial to life as we know it: the wrong-handed version of a drug molecule can be ineffective or even harmful.
Historically, chirality arises from covalent arrangements—how atoms are bonded together at asymmetric centers. But with mechanically interlocked molecules (MIMs) like catenanes, a new kind of chirality is possible. Instead of being baked into the covalent structure, chirality can emerge from the spatial arrangement of interlocked parts. This is known as mechanical chirality, and it’s changing how scientists think about molecular design.
Meet BPHC⁴⁺: Chirality Without the Bonds
A landmark study, recently published in Nature Synthesis, has introduced a catenane with tunable mechanical chirality, pushing the frontier of what’s chemically possible. The star of the study is BPHC⁴⁺, a compact, interlocked molecule born from an initially achiral compound, which—through clever molecular design—acquires chirality without altering its core covalent shape.
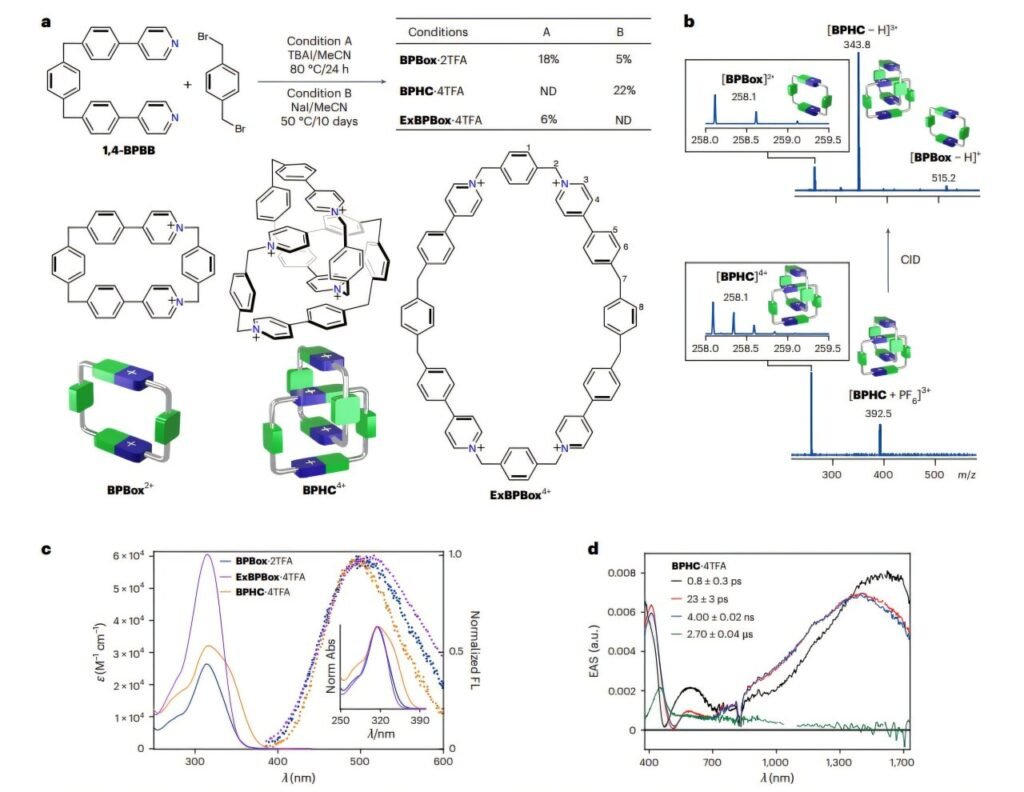
The innovation lies in how researchers introduced asymmetry mechanically, without disturbing the molecular skeleton. Instead of building chirality from inherently chiral pieces, they manipulated the way achiral components come together, creating chirality from the topological arrangement—like sculpting asymmetry out of a perfectly symmetrical rope simply by knotting it in a specific way.
From Symmetry to Asymmetry: Isostructural Desymmetrization
This groundbreaking approach relies on a strategy known as isostructural desymmetrization—a tongue-twister of a term that’s deceptively elegant in practice. Here’s how it works: imagine starting with a symmetric molecular ring that’s perfectly balanced—like a circle with mirror-image spokes. Now, subtly alter the spokes so they’re no longer identical, but don’t change the circular shape itself. The result is a molecule that looks the same from a distance but, under a molecular microscope, holds a secret asymmetry.
In this study, the researchers began with BPBox²⁺, a ring-like molecule similar to the well-known CBPQT⁴⁺ but tweaked for asymmetry. By replacing two identical bipyridinium units with monocationic phenylene-pyridinium groups, they lowered the molecule’s symmetry from C₂v (symmetrical over two mirror planes and a two-fold axis) to a less symmetric form. This reduction was enough to introduce mechanical chirality when two of these rings interlocked to form the catenane BPHC⁴⁺.
Why Chirality Matters in MIMs
The significance of this development goes beyond molecular novelty. It turns out that controlling co-conformational mechanical chirality—how the interlocked rings twist and turn relative to each other—opens the door to molecular switches. These tiny machines can flip between mirror-image forms, or enantiomers, without breaking any bonds. In the case of BPHC⁴⁺, this switch happens spontaneously at room temperature in solution, producing a racemic mixture—equal parts of both chiral forms.
But the researchers didn’t stop there. They introduced chiral disulfonate anions into the mix, which tipped the balance. Instead of two equal mirror images, one began to dominate, creating a measurable enantiomeric excess—in this case, about 18%. This is a powerful demonstration of induced chirality, where an achiral system becomes chiral under external influence.
Even more intriguingly, only the dominant enantiomer crystallizes from the solution. This points to a way of selectively extracting one chiral form—a crucial ability in pharmaceuticals, where one enantiomer of a drug may be therapeutic and the other inactive or harmful.
A New Chapter in Molecular Machines
The broader implications of this study are enormous. Molecular machines—nano-scale devices capable of movement and function—are a booming field of research, recognized with the Nobel Prize in Chemistry in 2016. These machines often rely on mechanical motion at the molecular level, and catenanes are one of their favorite building blocks.
By controlling chirality through mechanical means, scientists can now program molecular machines with an additional layer of functionality. Think of it like encoding handedness into a robotic arm—it can now distinguish between left and right, or perform different actions depending on its conformation. And since chirality plays a pivotal role in chemical recognition and reactivity, this adds a new axis of control for everything from drug delivery systems to responsive materials.
Why Compactness Matters
Another subtle but vital feature of BPHC⁴⁺ is its compact design. Large, floppy interlocked molecules tend to behave unpredictably. Their rings can slide or rotate freely, making it difficult to control their conformation. But compact catenanes, where the parts are tightly nestled together, offer much better conformational stability. That means more precise control over chirality and switchability.
BPHC⁴⁺ drew inspiration from an earlier compact catenane, HC8⁺, which had shown promise in locking mechanical conformations. But by using isostructural desymmetrization, the new molecule retained the compact benefits while gaining dynamic chirality—the ability to switch forms, yet settle into a preferred chiral state when prompted.
From Chemistry to Application: Why This Matters
So why should we care about catenanes with chirality that flips like a coin? Because this kind of chemical innovation is more than just a molecular parlor trick. It could revolutionize chiral drug synthesis, where selective production of one enantiomer can make or break a medication. It opens new avenues in sensing, where a molecule’s shape can determine how it responds to a target. It lays the foundation for molecular logic gates and switches that form the basis of future molecular-scale computing.
Furthermore, this study shows how chirality can be controlled without traditional covalent stereocenters. That’s a big deal. It means we can design molecules that act chiral without being built from inherently chiral pieces—saving synthetic complexity and opening new design pathways.
Looking Ahead: The Promise of Mechanical Chirality
As chemistry increasingly mimics the complex, dynamic behavior of biological systems, mechanical chirality will play an ever-greater role. Nature is full of chiral machinery—from enzymes to DNA—and now synthetic chemistry has a new tool that parallels this sophistication.
The work on BPHC⁴⁺ is a leap forward not just in molecular design, but in the philosophy of molecular architecture. It tells us that chirality isn’t just a static property—it can be dynamic, reversible, and induced. And, crucially, it can be designed with surgical precision from achiral pieces using the language of symmetry, mechanics, and topological cleverness.
As researchers continue to decode the hidden potential of interlocked molecules, catenanes like BPHC⁴⁺ may soon become staples of molecular factories, diagnostic tools, and drug libraries. The key to future breakthroughs might very well lie in the humble act of tying a molecular knot—with just the right twist.
Reference: Chun Tang et al, A compact catenane with tuneable mechanical chirality, Nature Synthesis (2025). DOI: 10.1038/s44160-025-00781-z
Loved this? Help us spread the word and support independent science! Share now.