For decades, astronomers have had a nagging cosmic mystery on their hands: more than half of the normal matter created in the Big Bang—known as baryonic matter—has gone missing. While dark matter and dark energy dominate the universe’s makeup, only about 15% of all matter is considered “normal,” or baryonic, composed of protons, neutrons, and electrons. But when scientists added up everything we can see—stars, planets, glowing gas—they could account for less than half of the expected baryonic matter. So, where did the rest go?
Now, thanks to an ambitious effort combining data from millions of galaxies and cutting-edge instruments, astronomers believe they’ve located this elusive material—not in stars or gas clouds, but in vast, diffuse halos of ionized hydrogen gas that encircle galaxies, stretching far beyond what we previously imagined. These halos form a nearly invisible scaffolding around galaxies, threading the universe with wispy filaments that are impossible to see directly, but detectable through subtle cosmic clues.
This groundbreaking discovery not only helps resolve a longstanding discrepancy in cosmology but also offers new insights into how galaxies evolve, how black holes shape their environments, and how the universe itself is structured.
The Missing Baryons Mystery
The story begins with the Big Bang, roughly 13.6 billion years ago. From this explosive beginning, the universe expanded and cooled, eventually forming hydrogen atoms—mostly protons and electrons—and a trace amount of helium. These became the building blocks of everything we see today: stars, galaxies, and interstellar gas.
Cosmologists have long relied on precise measurements of the cosmic microwave background (CMB)—the afterglow of the Big Bang—to determine the total amount of normal matter in the universe. The math is solid. Yet when they tried to count all that matter in the current universe, only about 30–40% could be accounted for in galaxies and hot gas.
The remaining 60–70% of baryonic matter was missing. Not in a metaphorical sense, but literally: it wasn’t appearing in any surveys of stars, dust, or visible hydrogen gas. This “missing baryon problem” was one of the most vexing issues in modern cosmology.
Hunting for the Invisible: A New Technique
To find something so faint and spread out that it’s practically invisible, astronomers had to get creative. The breakthrough came with the use of a subtle effect known as the kinematic Sunyaev-Zel’dovich effect (kSZ). It works like this: as the CMB photons journey across the universe, they can scatter off free electrons in ionized gas. If this gas is moving, the scattered photons are slightly redshifted or blueshifted, creating a minute change in the CMB’s brightness. This change is tiny—less than a millionth of a percent—but detectable with sensitive instruments.
Using this effect as a cosmic backlight, a team of researchers led by Boryana Hadzhiyska, a postdoctoral fellow at UC Berkeley, analyzed stacked images of nearly 7 million galaxies. By layering the data and amplifying the signal, they could detect the telltale signs of ionized gas far beyond what telescopes can directly observe.
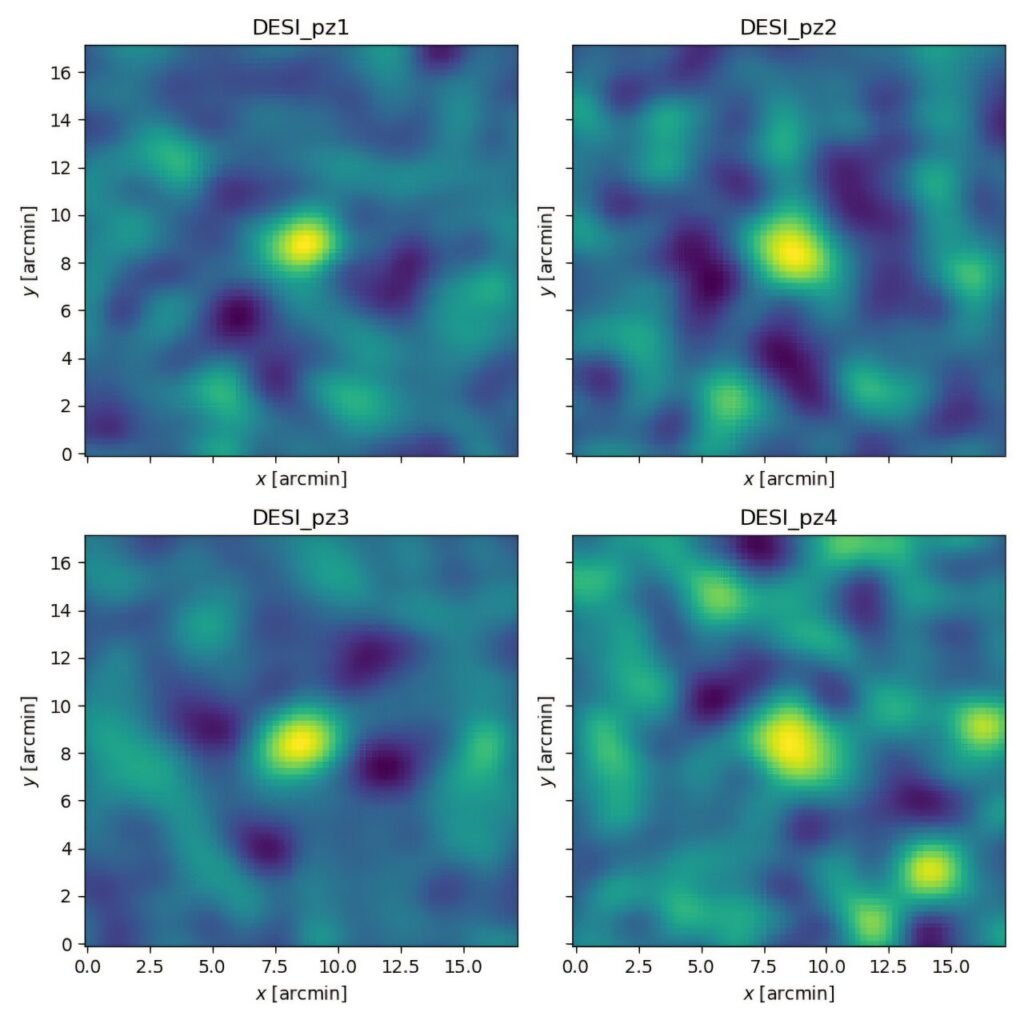
These galaxies came from the Dark Energy Spectroscopic Instrument (DESI) survey, while measurements of the CMB came from the Atacama Cosmology Telescope (ACT) in Chile. Together, the two datasets formed a high-resolution map of where this ionized gas might be hiding.
And the results? Striking. The gas wasn’t just hugging close to galaxies—it formed massive halos stretching five times farther than expected, blending into the cosmic web that connects galaxies across the universe.
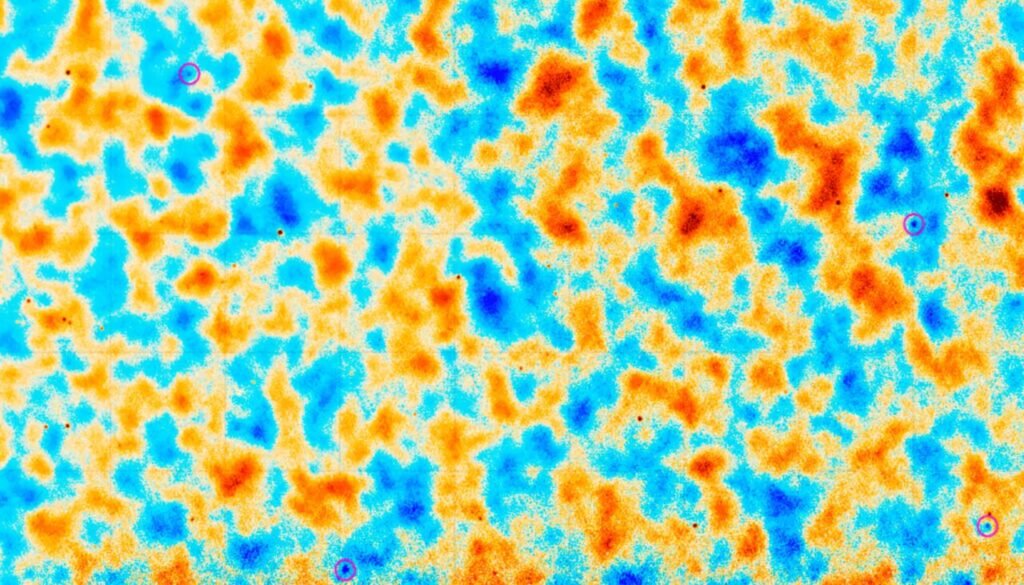
Black Holes: The Unexpected Architects
The presence of so much ionized gas far from galactic centers prompted another startling revelation: supermassive black holes might be more influential than we thought.
In the traditional view, black holes are active during a galaxy’s youth, spewing out jets of gas and radiation as they feed on infalling matter. These violent episodes are known as active galactic nuclei (AGN) phases, and they can temporarily halt star formation by expelling gas—the fuel for new stars—out of the galactic core.
However, the new study suggests these AGN may reignite periodically, pushing gas farther and more vigorously than current models predict. This cyclical on-off behavior is known as a duty cycle, and if AGNs flicker to life more often, they could be responsible for puffing up these extensive halos of ionized gas.
This process—known as feedback—is central to regulating galaxy evolution. When gas is pushed out by black holes, it cools over time and falls back in, creating a galactic fountain. This tug-of-war between gravity and feedback determines how many stars a galaxy forms, how bright it becomes, and how it changes over billions of years.
The Cosmic Web: Filaments and Halos
Another crucial aspect of the findings is the role of the cosmic web—the vast network of filaments connecting galaxies. These filaments, made of diffuse gas and dark matter, act like highways for galaxy formation. The newly discovered hydrogen gas doesn’t form a uniform sphere around galaxies; it aligns with these filaments, suggesting that matter distribution is even more complex than imagined.
This revelation supports earlier hints seen by Ferraro and Schaan, who analyzed similar signals in 2020. But the new dataset is far more robust, encompassing millions more galaxies and resulting in much higher precision. The findings were further confirmed by Bernardita Ried Guachalla, a Stanford graduate student, who used spectroscopic samples to show the uneven, filament-aligned distribution of gas.
Implications for Cosmology
Why does this matter? In cosmology, precision is everything. Modern theories of the universe’s evolution rely on assumptions about how matter—especially baryonic matter—behaves. If a significant portion of this matter is hiding in faint, extended halos rather than in galactic disks or stars, it changes how we interpret key observations.
For example, one of the open questions in cosmology is how “clumpy” the universe is—known as the sigma-eight problem. Current models sometimes conflict with observed clumpiness. If this missing gas is more widely spread, it could help smooth out inconsistencies.
It also impacts how we simulate the universe. Galaxy formation simulations must now factor in stronger feedback from black holes, more widespread ionized gas, and anisotropic gas distribution shaped by cosmic filaments. Some newer models already account for these factors, aligning more closely with the new observations.
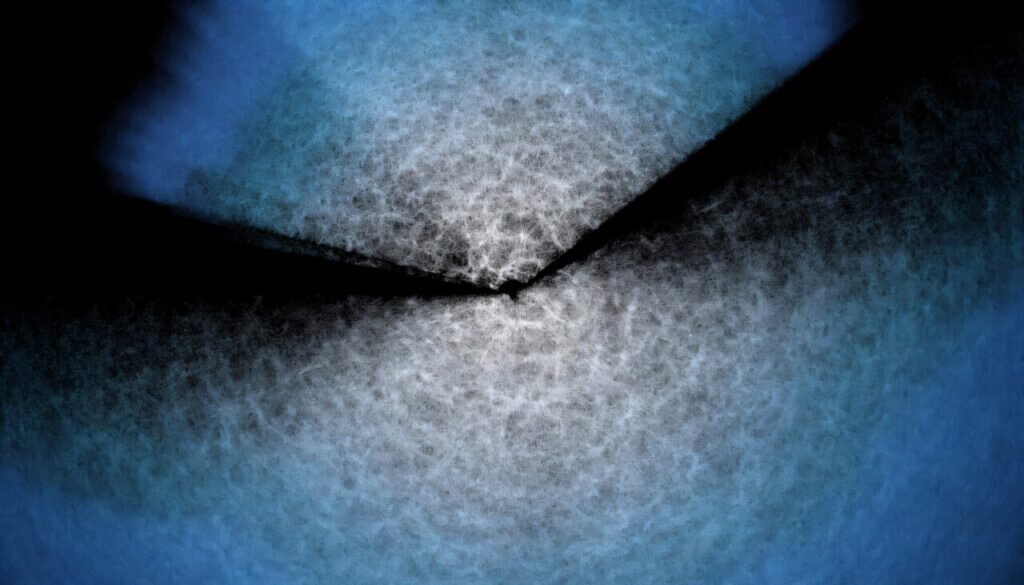
Looking Ahead: Probing the Early Universe
Perhaps the most exciting aspect of this research is what it unlocks for the future. The same techniques used to find this hidden gas—especially the kSZ effect—can be turned on the early universe, potentially allowing astronomers to study the infant cosmos and test fundamental physics, including general relativity and alternative theories of gravity.
As Hadzhiyska notes, the cosmic microwave background is “the edge of the observable universe.” By analyzing how it interacts with matter in various epochs, scientists can trace the structure of the cosmos over time, revealing how galaxies form, evolve, and interact with dark matter and dark energy.
A Collaborative Milestone
This discovery is a triumph of collaboration. The paper, co-authored by 75 scientists from institutions around the world, represents years of effort, spanning surveys like DESI and ACT, with contributions from Berkeley Lab, Stanford, SLAC, and others.
It’s also a testament to the power of innovative analysis: by stacking millions of faint signals, astronomers teased out a cosmic truth that had remained hidden in plain sight for decades.
Conclusion: A Clearer Map of the Cosmos
Finding the universe’s missing baryons is more than a checkmark on a cosmic inventory list—it’s a giant leap in understanding how the universe functions. These diffuse halos of ionized gas not only balance the baryon budget but also reshape our understanding of galactic feedback, black hole activity, and the web of matter that threads the cosmos.
As newer surveys launch and instruments grow more sensitive, this is likely just the beginning. We’re entering an era where even the faintest whispers of the universe can be heard—and from them, build a clearer, deeper map of the cosmos itself.
Reference: B. Hadzhiyska et al, Evidence for large baryonic feedback at low and intermediate redshifts from kinematic Sunyaev-Zel’dovich observations with ACT and DESI photometric galaxies, arXiv (2024). DOI: 10.48550/arxiv.2407.07152