Cell movement is fundamental to the development of multicellular organisms. Whether it’s the formation of tissues, the development of organs, or processes like wound healing, the ability of cells to move in a coordinated manner is essential. As an embryo grows, cell migration plays a key role in shaping its structure. However, until recently, scientists struggled to fully understand the complexities behind how cells know where to move and what triggers their collective migration. While much was known about chemical and mechanical cues that guide cells, recent research suggests that embryonic electrical fields also play an important role in guiding cells to their destinations.
A landmark study led by Dr. Elias H. Barriga, published in Nature Materials, has revealed critical findings about how bioelectric signals influence cell behavior during embryonic development. The study explores the fundamental role of electrical fields in guiding the migration of a specific group of cells known as the neural crest. This new evidence sheds light on the long-standing mystery of how cells in developing embryos respond to bioelectric signals and how they translate these signals into directional movements.
The Neural Crest and Its Significance in Embryonic Development
To appreciate the significance of the study, it’s necessary to understand the role of the neural crest in embryonic development. The neural crest is a population of cells that develops early during embryogenesis and migrates to form various structures in the body, including parts of the nervous system, bones, cartilage, and even components of the heart. Essentially, the neural crest is a highly versatile group of cells, contributing to multiple critical organ systems during development.
The development of the neural crest requires precise movements of cells to specific locations within the embryo. These movements have been primarily attributed to the signaling of molecular cues, such as growth factors and chemical gradients. However, the study by Dr. Barriga and his team uncovers a previously overlooked mechanism: the influence of electrical fields.
Electrotaxis: The Role of Electric Fields in Collective Cell Migration
Dr. Barriga’s team discovered that neural crest cells are directed by an endogenous electric field—a natural bioelectric current pattern generated within the developing embryo. This field acts like a road map, guiding cells to specific locations during embryonic development. The process in which cells follow these electrical cues is termed “electrotaxis.”
In essence, electrotaxis refers to the migration of cells in response to electric fields. This was a breakthrough, as the phenomenon had been observed primarily in cultured cells in laboratory settings, rather than in a living, developing embryo. The groundbreaking aspect of Dr. Barriga’s research was the demonstration of electrotaxis in vivo, meaning within the living embryo. The discovery suggests that electrical cues serve as more than just a backdrop for cell behavior—they are an active participant in driving cellular movement in a controlled and coordinated fashion.
The application of bioelectricity in directing cellular migration has significant implications, not only for understanding basic biology but also for applying that knowledge in medical fields like tissue engineering, regenerative medicine, and cancer research.
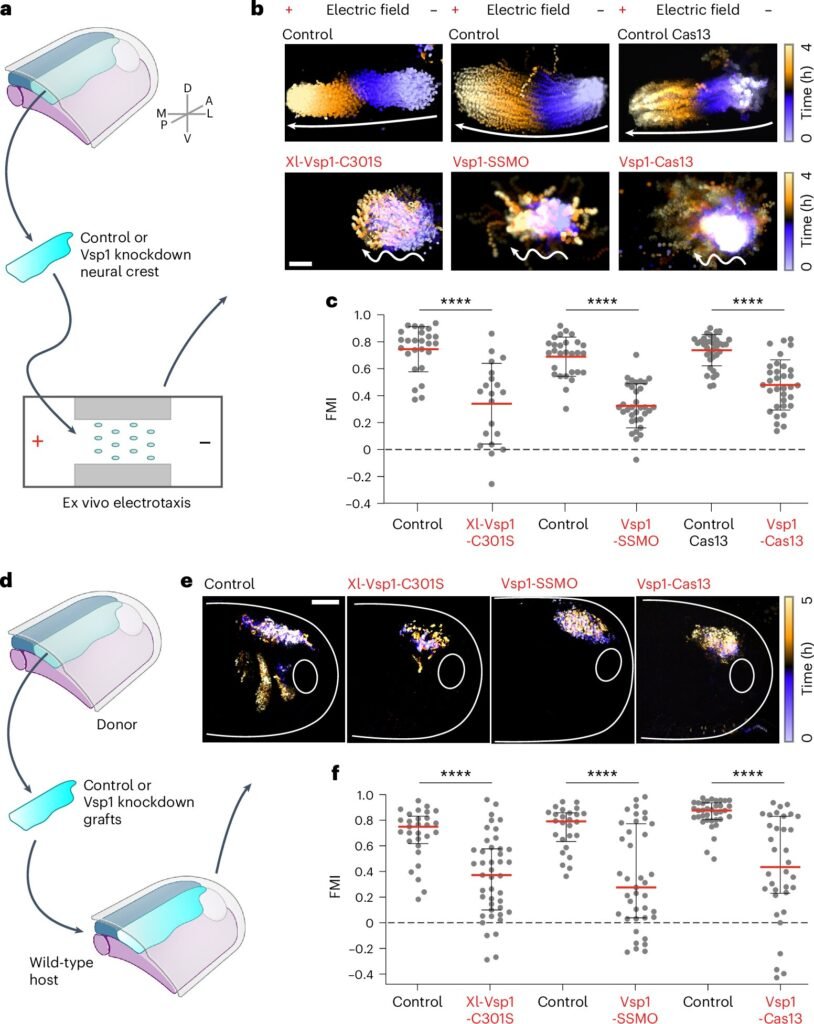
Understanding How Electrical Cues Guide Cells
While the study revealed that neural crest cells follow electrical cues, a crucial question remained: How exactly do cells interpret these electrical signals? To address this, Dr. Barriga and his team set out to identify the molecular machinery that cells use to sense and respond to electric fields.
The answer lay in the identification of a key enzyme, voltage-sensitive phosphatase 1 (Vsp1), in the neural crest cells. Vsp1 is uniquely equipped to sense the electric fields and help translate them into a directional signal. This process allows neural crest cells to orient themselves correctly and migrate toward their final destination in an organized manner. In a sense, Vsp1 acts as the ‘antenna’ that reads the bioelectric signals and informs the cells which way to move.
Dr. Sofia Moreira, a postdoctoral scientist and co-author of the study, remarked that targeting gene expression related to bioelectricity was a particularly rewarding aspect of the research. She highlighted the promise of these findings in advancing the understanding of bioelectric signals in development. The team’s experimental confirmation demonstrated that when a defective form of Vsp1 was introduced, collective cell migration impaired. This confirmed that Vsp1 plays a crucial role in electrotaxis.
Interestingly, Vsp1’s role is not to drive the physical movement of cells but rather to process electrical gradients and guide collective cell migration by indicating the proper direction. This distinction is noteworthy because, traditionally, enzyme sensors are thought to be involved in the actual movement of cells. However, Vsp1 seems to possess a unique function in guiding cells along a specific path in response to bioelectric signals.
How Electric Fields are Generated During Embryonic Development
An important part of Dr. Barriga’s study also uncovered how electric fields are generated in the first place. The research suggests that these electric fields arise through mechanical stretching of the neural fold, a region of the embryo crucial for the development of the neural crest. During this stretching, specific ion channels are activated, which leads to the establishment of voltage gradients. The voltage gradient essentially acts as an electric map that directs neural crest cells to migrate along predetermined paths.
When these voltage gradients are detected by Vsp1 in neural crest cells, the enzyme converts the electric signals into directional guidance, helping to orchestrate coordinated migration. The collaboration between mechanical forces and electrical fields in creating bioelectric patterns is an extraordinary example of the complexity involved in shaping the developing embryo.
The Importance of This Discovery for Future Research
This discovery is the first experimental demonstration that electric fields emerge along the neural crest’s migratory route, and it offers a detailed explanation of their origin and function. This insight deepens our understanding of bioelectricity’s pivotal role in orchestrating cell movement during embryonic development, providing new possibilities for mimicking these processes in laboratory settings.
By mapping how bioelectric fields guide collective cell migration, this research also opens new avenues for understanding how misregulation of such processes could lead to developmental disorders. Furthermore, this new knowledge could prove invaluable for areas such as tissue engineering, where controlling cell migration and organization is a significant challenge.
In the realm of regenerative medicine, understanding the role of electrical fields may lead to breakthroughs in stimulating the correct migration and integration of cells into damaged tissues. Similarly, insights gained from this work could help understand how electrical signals influence cancer cell movement, possibly contributing to the spread of tumors. The potential applications of this research stretch well beyond basic developmental biology, with implications for regenerative therapies and treatments of diseases.
Dr. Barriga expressed hope that these findings would serve as a valuable contribution to the scientific community’s understanding of developmental biology. However, there remains much more to explore. As Dr. Barriga pointed out, while the current study establishes that electrical cues play a critical role in guiding cell migration, further research is needed to integrate this new insight with existing models that emphasize the roles of mechanical and chemical signals during embryonic development.
A Broader Implication: Electrotaxis in Wound Healing and Cancer
Understanding the complex interplay of bioelectricity, cellular behavior, and movement might have profound implications for not just development but also for medical treatments. Similar electrotactic mechanisms may govern the behavior of cells in other contexts, such as wound healing or cancer metastasis. In wound healing, cells need to migrate to the site of injury, and manipulating the electric fields in the tissue could offer novel therapeutic strategies for accelerating repair. In cancer, cells can migrate away from primary tumors to form secondary growths, a process that is heavily influenced by electric signals. Unlocking how these signals influence disease progression might lead to innovative treatment methods.
Conclusion
The discovery that bioelectric fields play a crucial role in guiding cell migration during embryonic development represents a significant advancement in developmental biology. Dr. Barriga’s research, demonstrating how neural crest cells respond to electric fields through the enzyme Vsp1, bridges a long-standing gap in understanding collective cell movement. This groundbreaking finding not only enhances our knowledge of how embryos develop, but also opens new possibilities for advancing medical fields such as tissue engineering, regenerative medicine, and cancer research. By identifying how electric fields influence cellular behavior, the study provides insights into processes like wound healing and tumor spread. While much remains to be explored, this research offers a valuable foundation for future investigations into the interplay of mechanical, chemical, and electrical signals in shaping biological systems. The potential applications of bioelectricity in therapeutic interventions mark a promising frontier in regenerative medicine and disease treatment.
Reference: Fernando Ferreira et al, Stretch-induced endogenous electric fields drive directed collective cell migration in vivo, Nature Materials (2025). DOI: 10.1038/s41563-024-02060-2. www.nature.com/articles/s41563-024-02060-2
Think this is important? Spread the knowledge! Share now.