Geophysicists at ETH Zurich have recently made a groundbreaking discovery that could reshape our understanding of Earth’s internal structure and processes. Using advanced models of the lower mantle, the team has identified areas where earthquake waves behave differently than previously assumed, suggesting the presence of rocks that are colder or have a different composition than surrounding materials. These findings present a challenge to existing theories on the Earth’s plate tectonics and have opened up a major mystery in geophysical science.
The challenge in studying the Earth’s interior arises from the fact that no one can directly observe the interior layers—an area located miles beneath the surface—nor can we drill deep enough to obtain samples from the mantle. This layer of Earth lies between the core and the outermost rigid layer, the lithosphere. In the absence of direct measurements, geophysicists rely on indirect methods to probe deep beneath the Earth’s surface. One such method is seismic wave analysis, which uses earthquake recordings to infer what is happening within the planet.
In essence, the technique is similar to how doctors use ultrasound imaging to examine the interior of the body without making incisions. When earthquakes occur, seismic waves spread from the epicenter in all directions. As these waves travel through the Earth’s interior, they interact with various materials and are refracted, diffused, or reflected. The speed at which the waves propagate depends on factors such as the density and elasticity of the material through which they travel. This is where seismographs come into play—they record the different seismic wave behaviors as the waves pass through the Earth, allowing geophysicists to deduce the planet’s internal structure.
With this data, scientists have long been able to establish the structure of Earth’s interior, including the positions of submerged tectonic plates. These plates are located in areas known as subduction zones, where two tectonic plates collide and one is forced beneath the other into the Earth’s mantle. For years, this has been a key piece in understanding the dynamics of Earth’s plate tectonics and how plates are recycled back into the mantle. Subduction zones, where Earth’s crust is slowly consumed, have helped explain the cycle of plate tectonics and the planet’s geological history.
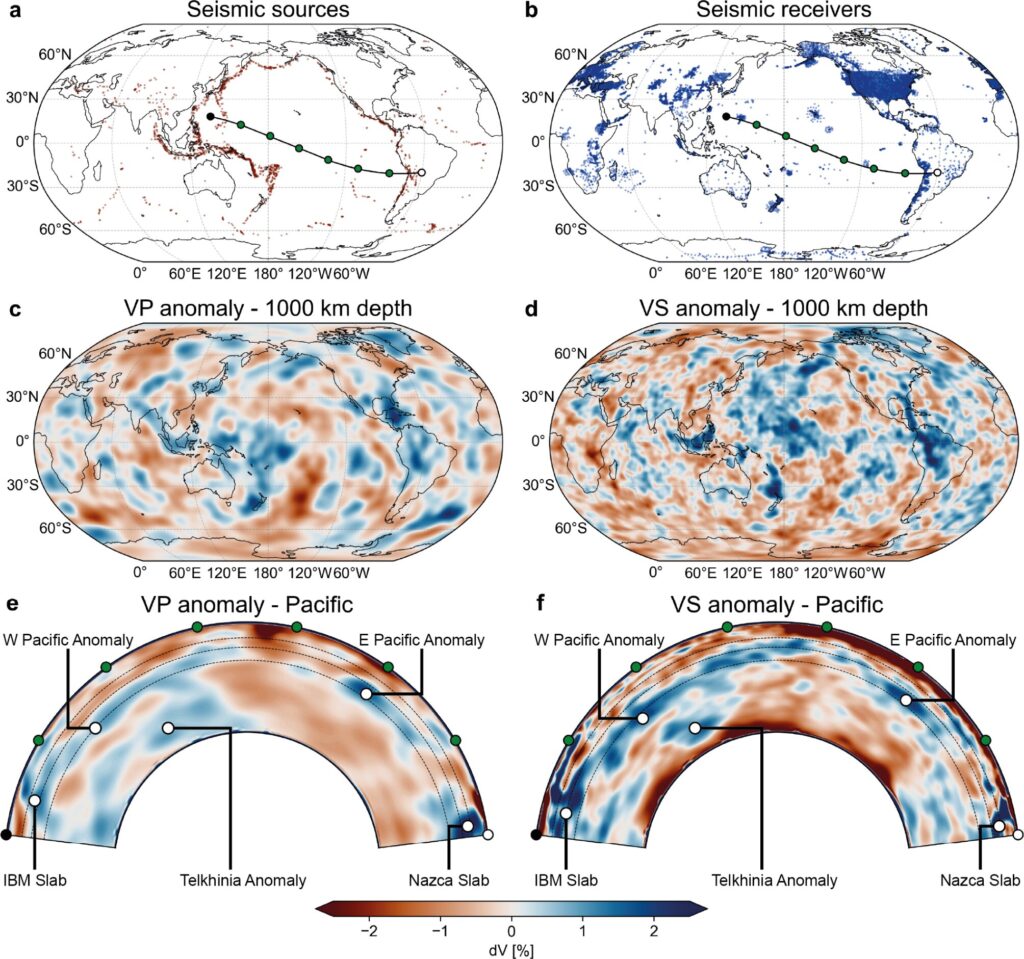
However, recent research by ETH Zurich and the California Institute of Technology has thrown a spanner into this long-held understanding. The team used a new high-resolution modeling approach to examine earthquake wave data more thoroughly than ever before. They discovered anomalies in the Earth’s mantle that look remarkably like submerged tectonic plates but are located far from expected subduction zones—areas that lie under oceans and even the interiors of continents, distant from any plate boundaries. Curiously, there is no geological evidence of subduction in these areas, raising questions about the composition and history of these peculiar regions.
The breakthrough is not based on a single seismic wave type but instead involves a comprehensive approach known as full-waveform inversion. This method analyzes all types of seismic waves—primary waves (P-waves), secondary waves (S-waves), and surface waves. It’s a computationally intensive technique that necessitated the use of the Piz Daint supercomputer at the Swiss Center for Scientific Computation (CSCS) in Lugano. By using this cutting-edge modeling tool, geophysicists could create a more accurate picture of the mantle’s structure, offering insights into the regions that traditional methods had overlooked.
The results are both intriguing and puzzling. According to Thomas Schouten, the doctoral student who led the study, these anomalies are far more widespread than previously thought, especially in the lower mantle. One significant finding is a previously unrecognized anomaly beneath the western Pacific—an area where, according to the current theory of plate tectonics, material from a subducted plate should not be present. Plate tectonic theories suggest that tectonic plates from this region should have either already been recycled or moved far away from this location in Earth’s geological past, leaving no traces.
The discovery of these unexpected anomalies raises more questions than answers. For now, the researchers are left speculating about the nature of the material responsible for these odd readings. Schouten suggests a few possible explanations. One hypothesis is that these regions contain ancient, silica-rich material that has been preserved in the mantle since the Earth’s formation about four billion years ago. This material could have endured the convective motions of the mantle over eons without being destroyed or redistributed. Another possibility is that the anomalies are due to areas where iron-rich rocks have accumulated as a result of mantle movements. Over the course of billions of years, these rocks may have gathered in certain locations and created the unique seismic wave patterns observed.
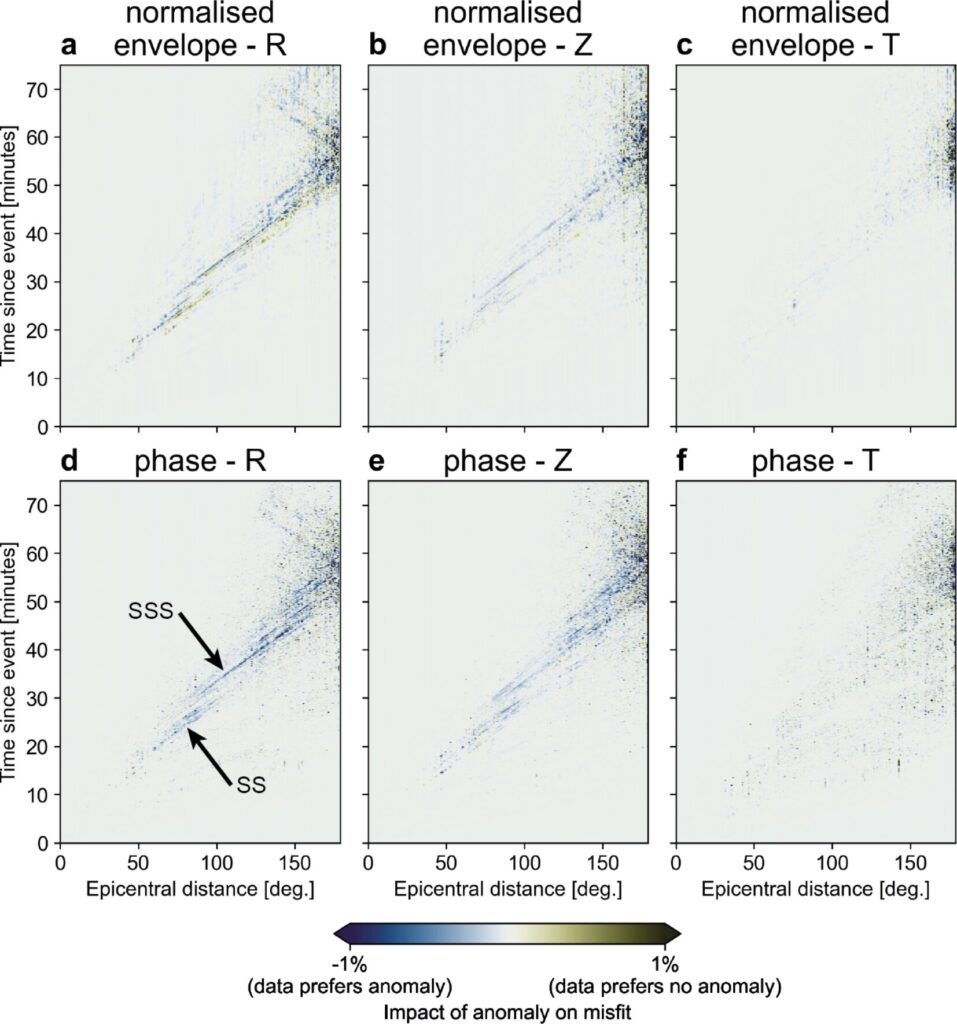
Professor Andreas Fichtner, who helped develop the computational model, likens the situation to a doctor using ultrasound for years to examine blood circulation and structures within the body. Until recently, they found everything where expected—organs, veins, and arteries in their usual positions. But now, with an upgraded tool, the doctor notices a new artery in an unusual location, an anomaly that isn’t quite explained by any current medical knowledge. This feeling of encountering the unexpected is what Fichtner and his team are experiencing in their analysis of the Earth’s interior.
At present, the team can’t definitively identify what material is responsible for the strange seismic activity detected in the lower mantle. The current models are focused primarily on the wave speed data, which, while useful, only provide a limited view of Earth’s complexity. As Schouten explains, seismic waves are essentially used to measure one property: the speed with which they travel through the Earth’s materials. However, this gives only a rough indication of what those materials might be, and more detailed models are necessary to uncover the physical characteristics behind the wave speeds.
The team’s next steps will involve refining the models and using other geological tools to better understand the anomalies they’ve uncovered. As Schouten points out, the waves analyzed in this model represent only one layer of information—they provide data on wave velocity but fail to explain the deeper properties, such as composition, density, and temperature. To get a more complete picture, the team will need to model multiple material properties that could explain the observed wave patterns. This further investigation could illuminate new details about how Earth’s deep layers operate and interact.
The study published in Scientific Reports is a call for more detailed and better models that could lead to a deeper understanding of Earth’s lower mantle. Such advancements could ultimately change how scientists think about Earth’s internal dynamics and might even offer new insights into the processes responsible for plate tectonics and the movement of material deep beneath the Earth’s surface.
In the end, this research could help reveal an entirely new chapter in our understanding of the planet’s geological history and deep inner workings. While the exact origins of the strange zones remain a mystery, the tools and techniques being employed by ETH Zurich’s team offer a promising future for geophysics and seismic research. By challenging long-established concepts, these findings encourage scientists to think beyond traditional paradigms and open the door to exciting new possibilities for studying our planet’s mysterious inner layers.
Reference: Thomas L. A. Schouten et al, Full-waveform inversion reveals diverse origins of lower mantle positive wave speed anomalies, Scientific Reports (2024). DOI: 10.1038/s41598-024-77399-2