Stars are born in vast clouds of gas and dust, and their early development remains one of the most intriguing yet elusive aspects of astrophysics. These clouds, which encompass material like hydrogen, helium, and heavier elements, are the nurseries where stars begin their journey. However, observing this process directly has proven difficult due to the dense and obscured nature of the birthplaces of stars, making it challenging to capture accurate real-time information about their formation. But recent advances in computational simulations have helped overcome some of these limitations.
One such breakthrough comes from a team of researchers at Chalmers University of Technology. They conducted high-resolution simulations that closely examine the mass-gathering process of a protostar, or a newborn star, with a mass similar to that of our Sun. This process, known as accretion, occurs when material from the surrounding circumstellar disk—a ring of gas and dust—is absorbed by the growing star. Accretion is a crucial step in the development of stars as it determines how much mass the protostar will eventually accumulate, affecting its size, luminosity, and eventual evolution.
The new simulations, which explore protostar evolution in unprecedented detail, are helping to answer some fundamental questions about the nature of accretion, especially how different factors influence the process. Specifically, the researchers focused on understanding the role of magnetic fields—an often overlooked but potentially critical factor in accretion. The results of this research were published in the renowned journal Astronomy & Astrophysics.
A Fundamental Question: How Do Protostars Accrete Mass?
The key question guiding the research by Brandt Gaches, an astronomer at Chalmers, and his team was centered around the mechanics of mass accumulation by protostars. The authors questioned how varying magnetic field strengths affected the process of accretion and whether those differences would lead to significant alterations in the formation of the star. Protostars, like the Sun, begin their lives within regions that are chaotic, often with material raining down from the circumstellar disk. Observations made through high-tech telescopes have contributed insights into the structure of protostellar systems, but theoretical models of how accretion happens during the early stages of stellar evolution remain somewhat underdeveloped.
In this context, Gaches and his collaborators undertook the challenging task of creating a simulation from scratch, without relying heavily on existing theories. They sought to simulate the behavior of a star with a mass equal to that of the Sun, focusing particularly on how magnetic fields impacted the accretion process. “For a protostar with the mass of the Sun, we wanted to understand how different strengths of magnetic fields would affect the material absorption process. Would there be a major change, or would the impact be marginal?” Gaches explained. This careful investigation opens new avenues for understanding the initial phases of a star’s life.
The Magnetic Field Factor
A significant aspect of the research focused on magnetic fields and their variable strengths, which can be crucial to how protostars grow. The magnetic field strength of young stars can vary considerably based on the material they gather from surrounding gas clouds. For example, newborn protostars can have weak or strong magnetic fields depending on the amount of magnetic material within their environments.
The way that these magnetic fields interact with the material of the surrounding disk can dramatically influence how gas accretes onto the star. Magnetic fields could influence the dynamics of gas flow, possibly obstructing or directing its flow towards certain regions of the protostar, such as the poles, instead of evenly distributing it across the star’s surface.
The research team modeled the behavior of four simulated protostars, each with the same mass as the Sun but different magnetic field strengths. These strengths ranged from 10 Gauss (roughly the same magnetic field strength as the Sun) to 2,000 Gauss, a value far exceeding that of the Sun. The simulations were designed to replicate the first two months in the life of a protostar, allowing the researchers to explore the physics at play during this pivotal stage of stellar evolution.
The results of these simulations revealed striking differences in the way gas is accreted depending on the magnetic field strength of the protostar.
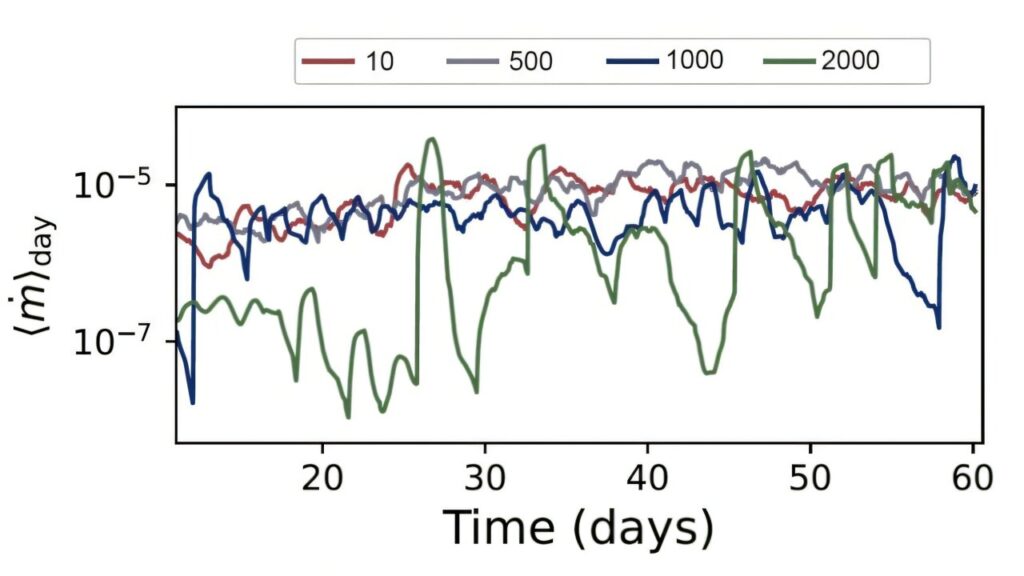
Variability in Accretion Rates: The Role of Magnetic Field Strengths
The key findings of the simulations provided important insights into the variability of accretion rates in stars with differing magnetic fields. In the case where the magnetic field was weak (around 10 Gauss), the accretion process was highly irregular and fluctuated rapidly. Gas flowed freely from the surrounding disk to the protostar in chaotic, uneven bursts. These large, unpredictable fluctuations were caused by the turbulent flow of the material, which resulted in sporadic accretion, with some intervals experiencing considerable increases in material absorption.
In contrast, when the magnetic field strength was increased to intermediate levels (500 and 1,000 Gauss), the material flow became more ordered. The increased magnetic fields helped organize the flow of gas, preventing the chaotic rainfall of material that occurred in the lower-magnetic case. This produced a steadier and less variable rate of accretion as the material was funneled in a more organized manner.
However, the most striking results came when the magnetic field strength was further enhanced to 2,000 Gauss. Here, the situation changed drastically. The magnetic field became strong enough to directly impact the star-disk interaction, disrupting the otherwise smooth flow of material from the disk to the protostar. In these simulations, gas no longer flowed uniformly across the star’s surface but followed a more structured path, adhering to the magnetic field lines instead. The material became funneled towards the protostar’s poles, concentrating the accretion in specific regions, with virtually no material escaping through the poles in this scenario.
Yet, one phenomenon appeared unexpectedly in the highly magnetized simulation: the sudden bursts of material piling up and then crashing onto the star’s surface. While the researchers anticipated a continuous, smooth flow of gas along the magnetic field lines, they observed that material would sometimes accumulate at the magnetic boundary, eventually building up enough pressure to lead to sharp, irregular bursts of accretion. These events contributed to spikes in the accretion rate and presented an interesting challenge to researchers, revealing how complex the relationship between magnetic fields and accretion can be.
Implications for Protostellar Research
Despite the different methods of accretion observed across the different magnetic field strengths, the total amount of material accreted in these scenarios showed little variation. Instead, the critical finding of the simulation was how the process occurred—ranging from chaotic to more orderly, punctuated by occasional bursts of accretion.
Interestingly, the simulations also revealed that the region of the star’s surface onto which the majority of the material falls remains constant across the different cases. Regardless of whether the magnetic field strength was weak or strong, the majority of the accretion happened on the star’s surface, without significant deviation in terms of the overall material inflow. This consistency is an intriguing aspect of protostar formation that warrants further exploration.
The team believes that their findings will provide essential clues for interpreting observational data about young stars, especially as scientists gather more information on the magnetic fields surrounding protostars. “We think these simulations motivate further observations of magnetic fields in protostars,” Gaches said. “Our simulations will help us interpret these observations and give us more tools to understand how stars like the Sun form.”
Looking to the Future of Protostellar Physics
These findings represent just the beginning of a broader investigation into star formation. While the study presented here provides new perspectives on how magnetic fields influence accretion, future work will focus on examining other aspects of protostar evolution. The team plans to expand these simulations to a broader range of conditions and for longer time periods to explore the full spectrum of physics at play during this critical early stage of stellar formation.
In the coming years, the high-resolution simulations generated by the Chalmers team could lead to significant advances in our understanding of how stars grow and evolve, addressing fundamental questions in astrophysics. As observational technology continues to advance, scientists will gain more accurate insights into the complexities of star formation, contributing to an even deeper understanding of the universe’s most fundamental processes.
Reference: Brandt A. L. Gaches et al, The High-resolution Accretion Disks of Embedded protoStars (HADES) simulations, Astronomy & Astrophysics (2024). DOI: 10.1051/0004-6361/202451842