Planet formation is a gradual process that spans millions of years. It begins when small dust and rock particles within a protoplanetary disk collide and stick together to form larger bodies. These collisions and their resultant accumulation of mass are known as accretion, a key process in the formation of planets. While accretion has been theorized for decades, it remains a subject of ongoing investigation. New insights into this process have been provided by a team of astrophysicists from the University of Duisburg-Essen (UDE), who conducted a series of groundbreaking experiments during a suborbital flight. Their findings, recently published in Nature Astronomy, offer vital new data on collision speeds and the electrical charge of the particles involved in planetary accretion.
The Birth of Planets: A Cosmic Evolution
The formation of a planet is not an instantaneous event but rather the result of a slow and steady accumulation of material over an extended period. It can take millions of years for a tiny dust grain, just micrometers in size, to eventually grow into a full-fledged planet several thousand kilometers across. The process begins within a protoplanetary disk, which surrounds a young star. This disk is composed mainly of gas (about 99%) with a smaller proportion of solid matter, primarily in the form of dust particles (approximately 1%).
These dust particles in the disk are not static; they are in constant motion, and collisions between them are inevitable. Upon colliding, dust particles stick together due to a process known as agglomeration, where smaller particles coalesce into larger masses. As these agglomerates continue to grow through successive collisions, they eventually form planetesimals, bodies between 1 and 100 kilometers in diameter. Through gravity, planetesimals can continue to attract surrounding material, gradually increasing in size until they become protoplanets, which may then evolve into fully developed planets.
The science behind this growth, however, has been far from clear-cut. One major challenge has been a physical barrier during the early stages of dust accumulation: Larger particles above about 1 millimeter in size tend to either bounce off one another or break apart due to the collision forces they experience. As a result, it has been difficult for dust grains larger than this size to grow into larger bodies. This phenomenon has been one of the most puzzling aspects of planetary formation. However, recent research from the UDE team, led by astrophysicists Prof. Dr. Gerhard Wurm and PD Dr. Jens Teiser, sheds light on how particles may still manage to grow beyond this “collision barrier.”
Collision and Charging: A Surprising Solution
A key insight from the UDE team is that dust grains, despite bouncing off each other at larger sizes, can overcome the collision barrier by acquiring electrical charges. “What’s fascinating is that collisions between these dust grains cause them to become electrostatically charged,” explained Prof. Wurm. “As a result, these grains can attract each other like tiny magnets, which leads to further agglomeration.” This phenomenon suggests that the electrostatic forces at play could help overcome the inherent problems of mechanical forces during collisions.
The team had previously observed this electrostatic adhesion in earlier laboratory experiments in drop towers, where gravity’s influence is minimized to simulate a microgravity environment. However, drop towers only allow for a brief 9-second window of microgravity, limiting the team’s ability to study the long-term evolution of the growing particles. For this reason, the UDE team decided to take their experiments a step further and utilize suborbital flights to achieve an extended microgravity environment.
Suborbital Experiments: A New Frontier for Astrophysics
In a bold and innovative move, the UDE team conducted their experiments aboard a sounding rocket launched by the European Space Agency (ESA). These rockets fly suborbitally, reaching altitudes of up to 270 kilometers. While ascending and descending, the rocket provides about six minutes of microgravity, which was just enough for the researchers to conduct a series of carefully designed experiments. During this time, the researchers were able to monitor the growth of agglomerates and measure the collision speeds with unparalleled precision, thanks to real-time controls and telemetry from the ground.
“We were able to directly observe the formation of agglomerates during this experiment,” said Dr. Teiser. “In a typical laboratory setting, we could never have studied such a process on this scale or in these conditions.” These agglomerates, formed through collisions between micron-sized dust particles, eventually reached sizes of about 3 centimeters—still far from planet-sized, but large enough to be highly significant for studying planetary growth processes.
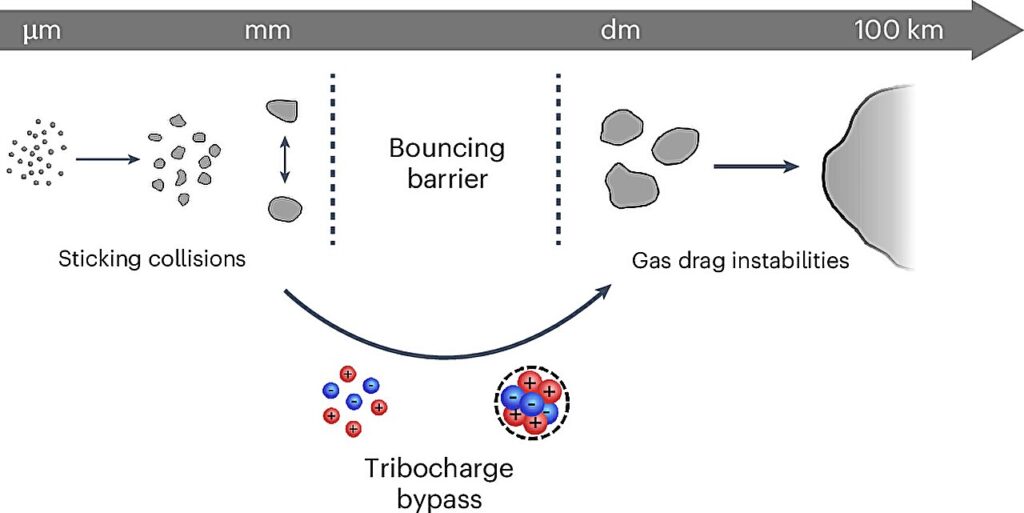
Key Discoveries: Stability and Erosion Speeds
Through this experiment, the UDE team made some crucial discoveries about how particle size and speed affect the stability of growing bodies. “We found that the agglomerates we observed could withstand collision speeds of up to 0.5 meters per second without eroding,” explained Prof. Wurm. “Anything faster, however, causes significant damage, disrupting the agglomerates and breaking them apart.” This is an important finding, as it gives a clear threshold for the maximum speeds at which dust particles can collide without destroying the building blocks of planet formation.
What surprised the researchers, however, was the specific speed at which erosion occurred. Previous models suggested similar speeds for when particles fragment, but to witness such a precise value in an actual experimental setup was a new revelation. The result confirmed that under the right conditions, these speeds directly correspond to the fragmentation speeds used in simulations designed to predict how dust particles break apart in a protoplanetary disk. These similarities suggest that the physical forces governing the growth and erosion of planetary bodies are, in fact, far more predictable than previously thought.
Dr. Teiser added, “We now know that when materials collide with speeds exceeding 0.5 meters per second, the outcome is fragmentation—an effect similar to what one might expect in the disk of a young star. This result reinforces our understanding that the conditions in the protoplanetary disk closely mimic those we observed in the laboratory.”
Electrostatic Charge and Attraction: A Powerful Driver
In addition to the insights on collision speeds, the UDE team’s experiments helped further confirm the role of electrostatic charge in particle attraction during accretion. Using numerical simulations, the team demonstrated that these electrostatic forces could indeed lead to stronger attraction between dust grains and, consequently, contribute to their agglomeration. As the particles collide and gain varying charges, the electrostatic attraction becomes a driving force that allows dust grains to cling together, overcoming mechanical barriers that would otherwise cause them to break apart.
“To understand how planets form, it’s crucial to look beyond just gravity,” said Dr. Wurm. “Our experiments show that electrostatic forces play a pivotal role in the initial stages of planet formation.” These findings not only address a gap in our understanding of the collision barrier but also open up new questions about how electrical forces can influence larger-scale processes in protoplanetary disks.
Implications for Planetary Science: Refining Models of Planet Formation
The results of these experiments are an essential addition to current physical models of protoplanetary disks and particle growth. These models now have the advantage of being able to incorporate experimental data on collision dynamics, erosion thresholds, and electrostatic effects. By incorporating these findings into simulations, astrophysicists can better predict the growth process of planetary bodies, gaining deeper insight into both the microscopic processes taking place within protoplanetary disks and the broader macroscopic evolution of planets.
The collaboration between experimentalists and modelers is crucial in modern astrophysics, and the findings from this research bring us closer to answering long-standing questions about how planets are born. From the electromagnetic forces at play to the exact conditions needed for particle growth to continue undisturbed, each new discovery helps to fine-tune our understanding of this complex and fascinating process.
Conclusion
The groundbreaking experiments conducted by the University of Duisburg-Essen team aboard a suborbital rocket have significantly advanced our understanding of planet formation. By examining the collision speeds and electrostatic charges involved in the accretion process, the researchers have provided vital insights into how dust particles within a protoplanetary disk can overcome barriers to growth and form larger bodies. Their findings reveal that agglomerates can withstand collision speeds up to 0.5 meters per second without fragmentation, providing a clearer understanding of the conditions required for planetary growth. Additionally, the role of electrostatic forces in attracting dust grains offers new perspectives on the forces shaping protoplanetary disks. These results are invaluable for refining existing physical models and offer a more accurate framework for understanding how planets are born. As future space missions continue to expand on this research, we edge closer to a complete understanding of planetary formation and the conditions necessary for life elsewhere in the universe.
Reference: J. Teiser et al, The growth of super-large pre-planetary pebbles to an impact erosion limit, Nature Astronomy (2025). DOI: 10.1038/s41550-024-02470-x. www.nature.com/articles/s41550-024-02470-x