In the dim, squirmy world beneath a rotting apple, a tiny fruit fly larva inches forward. It’s dark, damp, and teeming with invisible forces. No eyes to guide it. No ears to hear the world. But somehow, against all odds, it finds its way—drawn not by scent, sound, or taste, but by something far more mysterious: electricity.
Yes, electricity.
While we humans rely on sight, hearing, touch, taste, and smell to make sense of our surroundings, some animals boast a secret weapon: electroreception—the ability to perceive electric fields in their environment. Sharks use it to zero in on prey, bees use it to land on charged flowers, and the duck-billed platypus glides through murky water guided by electric cues.
Now, researchers at UC Santa Barbara have added an unlikely new name to this high-voltage VIP list: the humble fruit fly.
A Sixth Sense, Hiding in Plain Sight
It all started with a question. Matthieu Louis, a neurobiologist with a penchant for fundamental mysteries, had heard reports that the microscopic worm C. elegans could detect electric fields. That worm, like fruit flies, is a workhorse of biology labs. If worms could do it, why not flies?
Curious, Louis and his then-student Julia Riedl decided to test it. Not with complex equipment or elaborate tech—but with something so common in biology labs it might be collecting dust in a corner: a gel electrophoresis machine. Normally used to sort DNA, the device produces a stable electric field across a jelly-like slab. Instead of DNA, they dropped in fruit fly larvae.
And then the magic happened.
The larva wriggled, twisted, and made a choice. It turned toward the negative electrode and started crawling. Consistently. Predictably. Like it was following an invisible road sign.
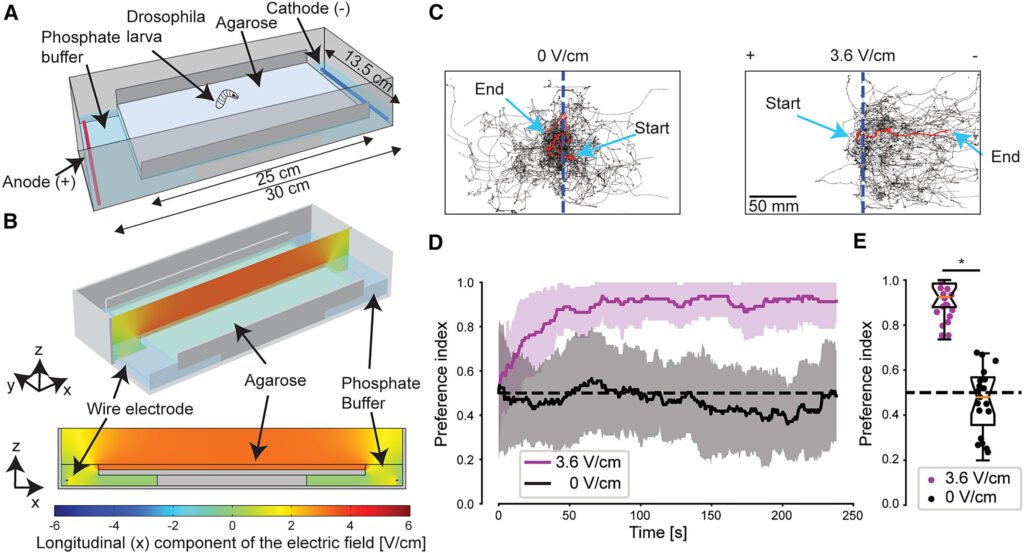
“I couldn’t believe it,” Riedl recalled. “The behavior was so robust. It wasn’t a fluke—it was like the larvae had been waiting all along for someone to notice.”
Searching for the Electric Brain
Sensing electricity is one thing. Figuring out how a creature does it? That’s another level of challenge.
The team had to reverse-engineer the larva’s nervous system—cell by cell—to find the culprits. They used a clever trick involving the gene GAL4, a favorite tool among geneticists. GAL4 acts like a switch, turning on other genes in specific neurons. By pairing it with a modified form of tetanus toxin (which silences neurons without killing them), the researchers could selectively shut down different parts of the larva’s brain.
Shut down neuron A—no change. Shut down neuron B—the larva stops reacting to electric fields. Bingo.
They pinpointed the source: a small cluster of neurons nestled in the larva’s head, near regions associated with smell and taste. To confirm the finding, they genetically modified the neurons to light up when active. Under the microscope, when an electric field hit the right spot, a single neuron flared like a lighthouse.
“It was beautiful,” Riedl said. “Just one neuron lighting up, and the whole behavior followed from that. Like flipping a switch.”
Even more stunning: the neuron responded differently based on direction. Field in front? Neuron shut down. Field behind? Neuron activated—and the larva spun around to face the negative end.
This wasn’t just detection. It was navigation.
A Puzzle 15 Years in the Making
You’d think such a thrilling discovery would rocket through academia in record time. But reality had other plans. This study was, in Louis’s words, “a scientific marathon.”
Launched in 2009 when Louis worked in Barcelona, the project dragged on for more than 15 years. Why? Because electric fields are notoriously tricky to study. Unlike magnetic fields (which iron filings can neatly reveal), electric fields are invisible, elusive, and easily muddled by confounding factors.
Could the larvae be responding to heat instead? Or changes in acidity? What about the current, rather than the field?
To get answers, Louis turned to collaborators outside the world of biology. Electrochemist Lior Sepunaru and mechanical engineer Alex Eden stepped in with modeling and simulation tools more at home in physics labs than biology departments. Eden’s models showed how electric fields formed inside the gel and how those fields interacted with the larva’s tiny body.
They fine-tuned the gel’s thickness, tweaked the solution’s chemistry, and even adjusted the electrodes. Eventually, they isolated the variable: the larvae were truly responding to the electric field—its direction, strength, and orientation—not to heat, not to acidity, not to random chance.
Why Would a Larva Need to Sense Electricity?
This discovery raises a deliciously strange question: Why would a squishy little maggot need electroreception in the first place?
One theory ties it to the larva’s natural habitat—fermenting fruit. As sugars break down and yeasts go wild, the resulting chemical soup creates subtle electric gradients. For a larva trying to survive its brief childhood, those gradients might point the way to richer, softer, less-alcoholic pulp.
Another theory takes a darker turn: predator avoidance. Flying insects, including parasitoid wasps, accumulate positive charges in the air. If Drosophila larvae learned to avoid areas of positive charge, they might dodge a gruesome fate—impaled by a wasp’s egg-laying needle. Given that up to 90% of larvae never reach adulthood due to such attacks, the stakes are high.
Or maybe it’s both. Evolution doesn’t need a single reason to grant a superpower—just enough benefit to make it stick.
The Electrosensory Enigma Deepens
Perhaps the most intriguing part of this whole affair isn’t just that fruit flies can sense electric fields—it’s how they do it.
In most electrosensitive animals, special mechanosensory organs detect the tiny movements caused by electric charge. It’s like how your hair might twitch near static. But the Drosophila larvae? No such apparatus has been found.
The active neuron is tucked inside a cluster normally used for taste and smell. It still reacts to bitter substances. Could the electric field be mimicking a bitter taste? That might explain the larva’s aversion to the positive pole—it “tastes” bad.
But this blending of senses opens up a Pandora’s box of questions. Could a neuron serve two roles—responding both to chemicals and electric fields? What molecular pathways allow this? And can this be harnessed for science?
Bigger Implications: From Healing to Biohacking
Why should we care if a fruit fly larva can read electric fields?
Because electricity is fundamental to life—not just in animal behavior, but in healing, development, and even cell migration. Our own cells respond to electric fields when forming organs or closing a wound. Cracking the genetic and cellular basis of electroreception in Drosophila might provide clues to these human processes too.
And that’s just the beginning.
In recent years, light-sensitive proteins from algae revolutionized neuroscience through optogenetics—controlling cells with pulses of light. Imagine a future version based on electric fields: a non-invasive, highly precise way to stimulate cells or alter gene expression.
“Studying a new sensory modality in a tiny Drosophila larva could open new directions for bioengineering,” Louis said.
From Curious Crawl to Scientific Shockwave
This discovery, humble as it began—with a larva crawling on a slab of gel—could become a cornerstone in our understanding of sensation, adaptation, and the wild possibilities of biology.
It’s a powerful reminder that nature never runs out of surprises. Even in a creature as tiny and well-studied as the fruit fly, there’s always more to uncover—hidden senses, ancient adaptations, and a quiet, electric story waiting to be told.
Reference: David Tadres et al, Sensation of electric fields in the Drosophila melanogaster larva, Current Biology (2025). DOI: 10.1016/j.cub.2025.03.014