A new study led by researchers at Kyushu University, Japan, has uncovered the important role of the three-nucleon force in nuclear stability, providing new insights that could have broad implications for our understanding of atomic structure, stellar processes, and the formation of heavy elements. The study, recently published in Physics Letters B, reveals how this specific force operates within atomic nuclei and how its effects grow stronger as the nucleus increases in size.
The Foundation of Nuclear Stability
All matter in the universe is made up of atoms, which are composed of a nucleus and surrounding electrons. The nucleus consists of protons and neutrons, collectively known as nucleons. These nucleons are held together in a stable arrangement by forces that govern their interactions within the nucleus. The most familiar of these forces is the strong nuclear force, which binds protons and neutrons together.
For decades, nuclear physicists have explored the nature of the strong force between nucleons, focusing largely on the two-nucleon force, which describes the interaction between two protons or two neutrons. The two-nucleon force has been studied extensively, leading to a solid understanding of how it contributes to the stability of atomic nuclei.
Tokuro Fukui, an Assistant Professor in the Faculty of Arts and Science at Kyushu University, points out, “While there’s significant understanding of the two-nucleon force and its role in nuclear stability, there’s less understanding of a more complex interaction — the three-nucleon force, in which three nucleons simultaneously interact with one another.”
The Two-Nucleon and Three-Nucleon Forces
In the realm of nuclear interactions, there are essentially two main forces at play. The two-nucleon force can be envisioned as a simple game of catch between two nucleons. The force carrier for this interaction is a subatomic particle called a meson, most notably a pion (the lightest of mesons), which mediates the long-range attraction between nucleons.
However, the story doesn’t end there. The newly investigated three-nucleon force involves the interaction between three nucleons, rather than just two. This force is more complex and involves the exchange of multiple pions between the three nucleons. Visualize it like a relay race where three nucleons “throw” pions to each other while simultaneously spinning and moving in orbit within the nucleus. This added complexity has made understanding the three-nucleon force a significant challenge.
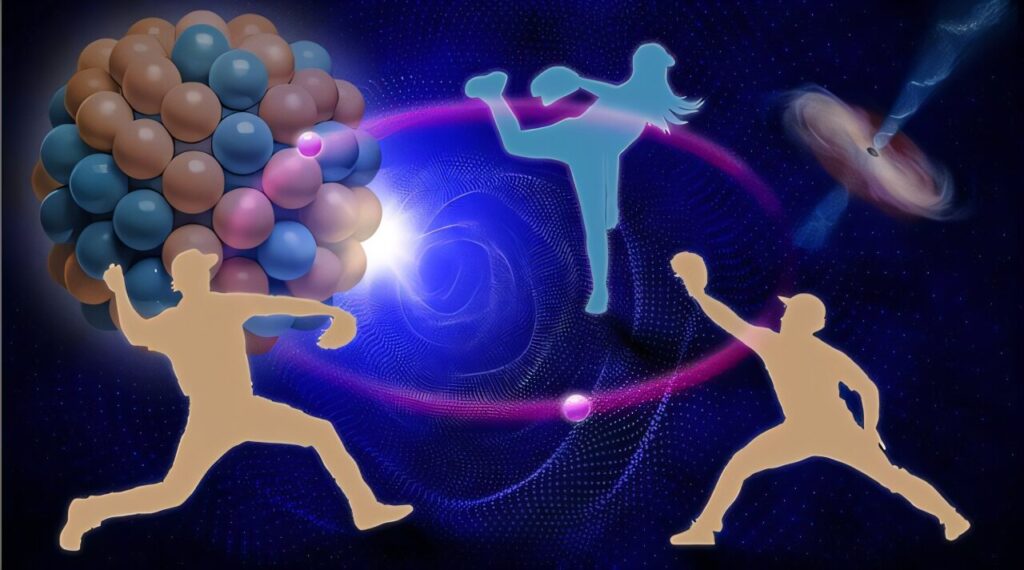
While it was once thought that the three-nucleon force wasn’t as crucial for nuclear stability compared to the two-nucleon force, this assumption has now been questioned. Recent research, including the current study, reveals that the three-nucleon force plays an unexpectedly significant role in enhancing nuclear stability — especially as the number of nucleons (protons and neutrons) increases.
New Insights from Supercomputer Simulations
To probe how the three-nucleon force affects nuclear stability, Fukui and his colleagues used advanced nuclear theory and supercomputer simulations to investigate how pions are exchanged between three nucleons. Their approach involved carefully modeling the interactions between pions and the resulting impact on the movement and alignment of nucleons.
In the simulation, the researchers discovered that when two pions are exchanged between three nucleons, the ways in which the nucleons move and spin become limited to just four possible combinations. This constraint proved crucial for understanding how the three-nucleon force contributes to nuclear stability.
Spin-Orbit Splitting and Energy Gaps
An important discovery made by the researchers is how the three-nucleon force enhances a process known as spin-orbit splitting. This concept refers to the interaction between a nucleon’s spin (how it rotates) and its orbital motion around the nucleus. When nucleons spin and move in the same direction, it results in a lower energy state. Conversely, when nucleons spin in the opposite direction to their orbital motion, they end up in a higher energy state.
Fukui explains, “Our simulations showed that the three-nucleon force not only increases the energy of nucleons with aligned spins and orbits, but it increases the energy of nucleons with opposing spins and orbits to an even greater extent. This results in a significant energy gap between different energy shells in the nucleus, thus making it more stable.”
This energy gap creates a kind of stabilizing shell structure within the nucleus, where nucleons occupy specific energy levels. When the nucleus has a stable energy gap between shells, it becomes much harder for the nucleus to absorb more nucleons or neutrons, which plays a crucial role in the formation of heavy elements.
The increased strength of the three-nucleon force leads to larger energy gaps between nucleon shells in heavier nuclei. This effect becomes particularly significant as the nucleus increases in size. For example, in carbon-12 — a relatively light but essential element with 12 nucleons — the researchers found that the three-nucleon force caused the energy gap to increase by a factor of 2.5 compared to what would be expected from the two-nucleon force alone.
Fukui points out, “This effect is so large that it’s nearly on par with the two-nucleon force, and we anticipate that it will be even stronger for heavier elements. This finding sets the stage for further studies on larger atomic nuclei.”
Implications for Star Chemistry and Element Formation
One of the most important aspects of this research is its potential to illuminate how heavy elements are formed in the universe, particularly in stars. Nucleosynthesis, the process by which new atomic nuclei are created, takes place primarily through nuclear fusion in stars. In the cores of stars, light elements like hydrogen and helium fuse together to form heavier elements. The newly discovered role of the three-nucleon force provides important clues about this process.
As the three-nucleon force strengthens in larger nuclei, it increases the energy gaps between nuclear shells. This added stability means that larger nuclei are more resistant to capturing additional neutrons. In turn, this resistance to further neutron absorption complicates the creation of elements heavier than iron — a key limitation in stellar nucleosynthesis. Additionally, certain nuclei containing “magic numbers” of protons or neutrons, such as carbon-12, become so stable that they can act as building blocks in further fusion processes.
Fukui adds, “The understanding of these energy gaps is essential for predicting how the heavier elements form in stars, and the three-nucleon force is a central piece of this puzzle. In particular, magic number nuclei are highly stable and could pose barriers to forming heavier elements in the stellar interiors.”
Quantum Entanglement and Future Implications
The study also revealed a surprising consequence of the three-nucleon force: the creation of quantum entanglement between nucleons. In systems involving only the two-nucleon force, each nucleon’s spin is measured individually. However, when the three-nucleon force comes into play, it leads to a quantum entanglement phenomenon, where two of the three nucleons exist in both spin states at once until measured.
This unusual behavior could have profound implications for future research in both nuclear physics and emerging technologies such as quantum computing. The concept of quantum entanglement has already revolutionized fields like quantum mechanics and computing, and this discovery suggests that similar entanglement might occur within atomic nuclei, though with differences in behavior due to the larger mass of nucleons compared to other particles like electrons.
Fukui concludes, “The entanglement of nucleons is a fascinating result, as it might offer new insights into the quantum properties of atomic nuclei. Given the emerging interest in quantum computing, this discovery may open the door to applications in quantum technologies.”
Conclusion: Strengthening Our Understanding of Nucleon Forces
This new research by Kyushu University marks a significant step forward in understanding the dynamics of nuclear stability. By revealing the importance of the three-nucleon force and its impact on energy gaps, nuclear shells, and nuclear stability, the findings not only advance our theoretical understanding of atomic structure but also offer new directions for studying the formation of elements in the universe. With these insights, we move closer to deciphering the processes that power the stars and produce the elements necessary for life.
Reference: Tokuro Fukui et al, Uncovering the mechanism of chiral three-nucleon force in driving spin-orbit splitting, Physics Letters B (2024). DOI: 10.1016/j.physletb.2024.138839