Quantum mechanics continues to push the boundaries of human understanding, revealing bizarre and counterintuitive behaviors in materials at the smallest scales. Among the most fascinating phenomena observed in quantum materials are fractional quantum Hall (FQH) states. These states emerge under specific conditions, such as the presence of a strong magnetic field at ultra-low temperatures, and exhibit unique properties, including the potential for new types of particles that could form the basis of topological quantum computers.
Traditionally, scientists have used resistivity measurements—assessing how much a material resists the flow of electric current—as the primary tool to detect and explore FQH states. However, new research by a team of physicists at George Mason University, in collaboration with Brown University and the National Institute of Standards and Technology (NIST), is challenging the conventional approach. Their study, published in Nature Physics, demonstrates that thermopower measurements—quantifying the voltage generated when heat is applied to a material—can provide more precise insights into these elusive quantum states, especially in bilayer graphene.
Understanding Thermopower: The Link Between Heat and Voltage
Before diving into the specifics of the research, it is essential to understand the concept of thermopower. When one side of a conducting or semiconducting material is heated while the other remains cool, the resulting temperature difference causes charge carriers (such as electrons) to move from the hot side to the cold side, creating a voltage difference. This phenomenon is known as thermopower, or the thermoelectric effect.
In simple terms, thermopower is the voltage that arises from a temperature gradient in a material. For years, scientists have known that thermopower is closely linked to the entropy of a system—the degree of randomness or disorder at the microscopic level. The more entropy a system has per charge carrier, the greater the thermopower it produces. This relationship allows researchers to probe materials at a deep thermodynamic level, offering insight into the behavior of charge carriers and the underlying physics of the material.
The Fractional Quantum Hall Effect and Its Importance
The FQH effect, a quantum phenomenon first observed in 1982, occurs in two-dimensional electron systems subjected to a strong perpendicular magnetic field at extremely low temperatures. In these systems, electrons form collective behaviors that lead to the formation of quasiparticles—particles that behave differently from single electrons. These quasiparticles can exhibit fractional charges, a concept that defies traditional notions of particle physics. The study of FQH states has since become a focal point in condensed matter physics, with the potential to uncover new particles and exotic quantum phases of matter.
In addition to being a subject of academic intrigue, FQH states have profound practical implications. Some of the quasiparticles that arise in these states are believed to be non-abelian anyons, particles whose properties make them ideal for use in topological quantum computing. Topological quantum computers rely on the unique properties of these particles to perform computations in ways that are more stable and resistant to errors than conventional quantum computers.
For years, the primary method for detecting and studying FQH states was by measuring the resistivity of the material. However, this method has its limitations, particularly in the case of fragile FQH states that may not produce significant resistive signatures. This is where thermopower comes into play.
A Shift in Approach: Using Thermopower to Detect FQH States
The research team, led by Dr. Fereshte Ghahari, recognized the potential of thermopower measurements to reveal properties of quantum states that resistivity measurements could miss. Ghahari and colleagues aimed to explore how thermopower could serve as a more sensitive and reliable method for detecting fragile FQH states, particularly in materials like bilayer graphene.
Bilayer graphene, a material made up of two layers of carbon atoms arranged in a specific structure, has emerged as an exciting platform for studying quantum phenomena. The two-dimensional nature of bilayer graphene, coupled with the ability to tune its electronic properties, makes it an ideal candidate for exploring exotic quantum phases like the FQH effect.
Previous research on bilayer graphene focused on resistivity measurements to detect FQH states. However, this approach only revealed certain states and missed others that may have been present in the system. Ghahari and their collaborators sought to address this gap by using thermopower to probe the entropy of the system, which would offer more detailed insights into the behavior of charge carriers in the presence of FQH states.
The Experiment: Applying Thermopower to Bilayer Graphene
In their groundbreaking study, Ghahari and their team used thermopower-based measurements to analyze the quantum properties of Bernal-stacked bilayer graphene—a specific arrangement of graphene layers that exhibits unique electronic behavior. They applied a temperature gradient across the material and measured the resulting voltage, allowing them to capture the thermopower signal associated with the FQH states.
The results were striking. Not only did thermopower measurements reveal fragile FQH states that had previously been undetected using resistivity, but they also uncovered entirely new FQH states that had not been reported before. These new states appeared in the thermopower signal, offering new clues about the underlying physics of the system.
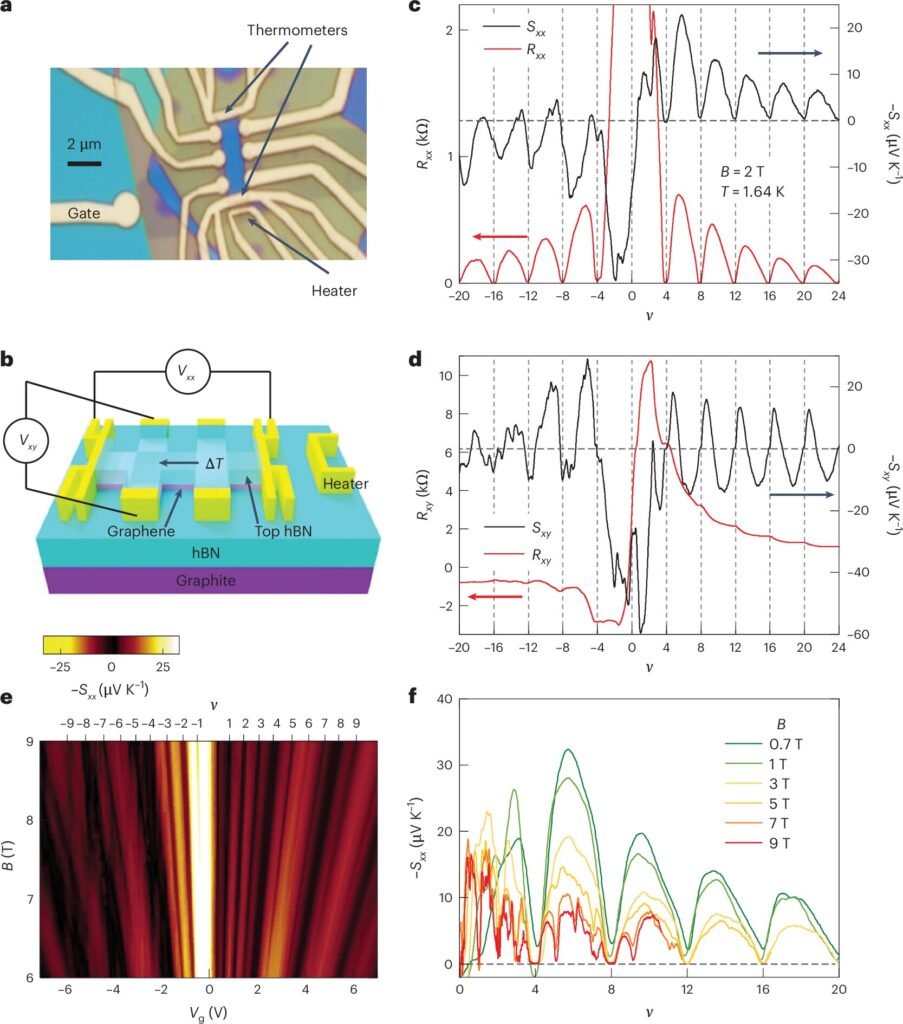
This discovery opens up a new avenue for studying strongly interacting quantum phases, particularly in materials like bilayer graphene. By using thermopower as a tool, researchers can now probe the entropy and behavior of charge carriers with greater sensitivity, enabling a more detailed understanding of FQH states and other exotic quantum phenomena.
Implications for Quantum Computing
The implications of this research extend beyond just academic interest. As Ghahari explains, some of the new particles that emerge in FQH states may serve as the building blocks for topological quantum computers—an emerging field in quantum computing that seeks to harness the unique properties of non-abelian anyons to perform computations. These particles are highly resistant to noise and errors, making them a promising candidate for developing more stable and scalable quantum computers.
Topological quantum computers rely on the manipulation of these exotic quasiparticles, and understanding how they behave is essential for advancing this technology. By using thermopower measurements to better detect and study FQH states, the research team is laying the groundwork for future investigations into the potential applications of these states in quantum computing.
The Future of Thermopower in Quantum Research
Ghahari’s work represents just the beginning of what could be a transformative shift in how we study quantum materials. The ability to use thermopower to detect fragile quantum states opens up new possibilities for both theoretical and experimental research. This approach could be applied to a wide range of materials, including moiré materials, where periodic patterns of atomic layers can create new electronic behaviors.
Additionally, the combination of thermopower measurements with other experimental techniques, such as quantum Hall effect measurements and scanning tunneling microscopy, could provide a more comprehensive view of the complex interactions within quantum materials.
As researchers continue to refine thermopower-based techniques, we may see even greater advancements in our understanding of strongly interacting quantum phases of matter. These breakthroughs could lead to the development of novel quantum devices and technologies, including topological quantum computers, that could revolutionize the way we process information in the future.
Conclusion: A New Era in Quantum Phase Exploration
The work by Dr. Ghahari and their team represents a significant leap forward in the study of quantum materials, particularly in the detection and understanding of fractional quantum Hall states. By leveraging thermopower measurements, they have opened new avenues for exploring the enigmatic properties of strongly interacting quantum phases and their potential applications in quantum computing.
As the field of quantum physics continues to evolve, the findings of this study will undoubtedly inspire further research and experimentation, bringing us closer to realizing the promise of topological quantum computers and other groundbreaking quantum technologies. The use of thermopower to probe the entropy and behavior of charge carriers is just one example of how innovative approaches in experimental physics can lead to profound advancements in our understanding of the quantum world.
Reference: Nishat Sultana et al, Detection of fractional quantum Hall states by entropy-sensitive measurements, Nature Physics (2025). DOI: 10.1038/s41567-025-02813-z.