Quantum computing is no longer just a futuristic concept confined to theoretical discussions and speculative projections. It’s evolving into a tangible, transformative technology capable of solving some of the most complex problems in science and engineering. And while quantum supremacy—when quantum computers outperform the best classical supercomputers in a specific task—was first achieved through tasks like random circuit sampling, researchers are now setting their sights on a much more consequential frontier: simulating the true, continuous-time dynamics of quantum systems that underpin the laws of nature.
In a remarkable step forward, researchers from Quantinuum and collaborating institutions across Europe and the U.S. have done just that. They used a powerful quantum processor, known as H2, to simulate the digitized time evolution of the quantum Ising model, a cornerstone in the study of quantum magnetism and many-body physics. The simulation didn’t just replicate a well-understood phenomenon—it ventured into territory classical computers struggle to reach. In this exploration, they captured a fleeting, exotic phenomenon called Floquet prethermalization—a transient state where systems appear locally stable before relaxing into equilibrium.
This marks a milestone in quantum simulation, showcasing that quantum computers are beginning to operate in regimes that classical methods can barely touch, let alone conquer.
What Makes Quantum Computers Special?
To understand the impact of this achievement, we must first grasp the inherent strength of quantum computers. Classical computers process information in binary—bits represented as either 0 or 1. Quantum computers, however, use qubits, which can exist in a superposition of both 0 and 1 simultaneously. Add to this entanglement, where the state of one qubit can instantly influence another, and interference, where probabilities are manipulated for desired outcomes, and you have a machine fundamentally different from anything classical computing offers.
While classical systems falter under the weight of exponential complexity, quantum systems thrive. This makes them especially suited for simulating quantum materials, high-energy particle systems, and even the behavior of the early universe.
But here’s the catch: building reliable quantum computers that can simulate continuous processes in nature is immensely difficult. Simulating time-dependent quantum systems requires more than just having qubits—it demands precision control, low error rates (high fidelity), and scalable architecture.
Quantinuum’s recent results show that this is no longer a distant goal, but an emerging reality.
The Quantum Ising Model: A Playground of Magnetism and Phase Transitions
The quantum Ising model isn’t just a toy problem for physicists. It’s a foundational framework used to explore quantum phase transitions, magnetic ordering, and emergent behaviors in complex materials. The model describes particles (or spins) arranged in a lattice, with interactions between them dictated by quantum mechanical rules.
What makes this system particularly rich is its non-equilibrium behavior. When pushed far from equilibrium—say, by a sudden change in an external field or internal parameters—the system doesn’t instantly settle into a new equilibrium. Instead, it undergoes complex transitions, exhibiting intermediate states that can be incredibly hard to capture and describe. Floquet prethermalization is one such state—essentially a temporary plateau where the system behaves as though it has reached equilibrium, only to later evolve further.
This intermediate “false equilibrium” is fascinating, not just because of its theoretical implications, but because it’s relevant in understanding thermalization in quantum systems, and even how energy might propagate in novel materials or engineered quantum environments.
Capturing this behavior digitally on a quantum computer is no trivial feat. It requires simulating smooth, continuous time evolution—a fundamental property of real-world physics—using the discrete building blocks of quantum gates.
Digitizing the Continuum: From Smooth Time to Quantum Gates
“In real systems, time passes smoothly,” said Dr. Michael Foss-Feig, lead researcher at Quantinuum. “Simulating realistic dynamics on a digital quantum computer requires ‘discretizing’ this smooth time evolution into the set of quantum gates that one has available.”
In simple terms, simulating natural dynamics on a quantum computer means slicing smooth processes into step-by-step gate operations. It’s like trying to recreate a flowing river using LEGO bricks. Done poorly, and the simulation looks jagged and inaccurate. But with high enough resolution and precise control, the digital approximation becomes nearly indistinguishable from the real thing.
That’s exactly what Quantinuum achieved. Their H2 system demonstrated gate fidelities high enough to sustain coherent, low-noise simulations over long enough timescales to capture emergent behavior, like the onset of hydrodynamic energy transport and prethermal plateaus—features that classical simulations can barely approach without vast computational resources.
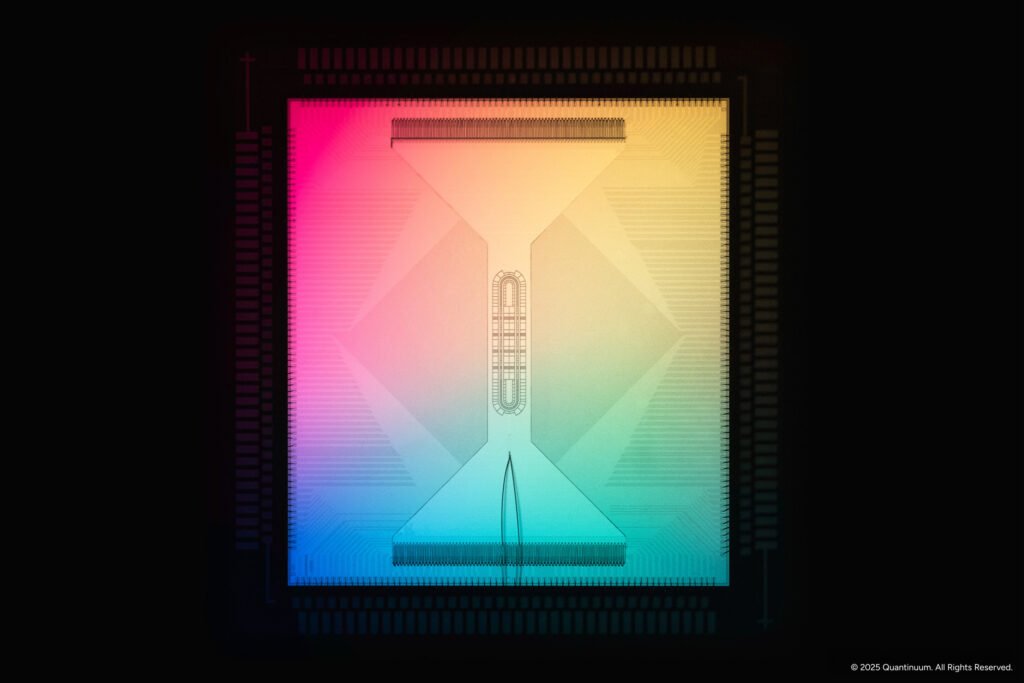
Classical Struggles and the Edge of Quantum Advantage
One of the major challenges in theoretical physics is computational intractability. As systems grow in size or complexity, classical methods (even the most sophisticated tensor networks or Monte Carlo simulations) begin to falter. Every added particle, interaction, or time slice increases the required memory and processing exponentially.
Reza Haghshenas, who led the benchmarking efforts, elaborated: “The most important achievement of this work is that we were able to keep this continuous time-evolution relatively noise-free (and therefore accurate) out to timescales where even the best classical simulation methods appear to really struggle.”
While it might still be technically possible to reproduce similar simulations on a supercomputer, the cost is enormous—demanding days of runtime, massive memory, and specialized algorithms, just to match a quantum machine running in real-time with only 56 qubits.
The takeaway? Quantum simulation is beginning to offer a genuine computational edge in real-world physics, not just contrived tasks like random circuit sampling.
From H2 to Helios: Scaling Up the Quantum Horizon
Quantinuum’s H2 machine is already powerful, but the team isn’t stopping there. They’re preparing to release Helios, their next-generation system featuring 96 qubits. According to Foss-Feig, this step up in scale is expected to push simulations into regimes that are undeniably out of reach for classical approaches.
“Moderate increases in system sizes should enable simulations that are more definitively out of reach of current classical methods,” he explained. “We are very much looking forward to releasing Helios.”
Why does this matter? Because as the number of qubits grows, the quantum system can model larger, more complex physical systems—like strongly correlated electron materials, exotic quantum phases, and dynamics relevant to high-energy particle physics or condensed matter.
And unlike classical simulations, where each added particle might double or triple the workload, quantum computers handle the extra complexity natively. It’s part of their design.
The Future: A New Era of Scientific Discovery
What makes this breakthrough particularly exciting isn’t just the immediate scientific gain—it’s the broader implications.
Many of the greatest technological revolutions of the past century stemmed from our ability to simulate quantum systems: transistors, lasers, semiconductors, magnetic materials. Yet these were derived from a limited, approximate understanding of quantum behavior. Imagine what becomes possible when we can simulate these systems accurately, at scale, and in real-time.
From designing high-temperature superconductors, to uncovering new states of matter, to probing the boundaries of quantum gravity—quantum simulation could become the bedrock of 21st-century physics and engineering.
As Foss-Feig put it, “Quantum computers may soon be capable of validating and augmenting classical simulations in settings where classical methods are infeasible or unreliable.”
Final Thoughts: The Dawn of Real Quantum Science
For decades, physicists dreamed of quantum computers not just as faster calculators, but as new kinds of laboratories—capable of recreating the universe’s most complex phenomena in a box. Quantinuum’s success with simulating the quantum Ising model, and capturing phenomena like Floquet prethermalization, marks a turning point toward that vision.
We are witnessing the dawn of a new era—not just in computation, but in how science itself is done. With each new qubit, each refinement in fidelity, quantum computers are expanding the frontiers of what we can explore, understand, and ultimately create.
And while the road ahead still holds engineering challenges and theoretical puzzles, one thing is clear: the future of quantum simulation is no longer theoretical.
It’s here. It’s now. And it’s only just beginning.
Reference: Reza Haghshenas et al, Digital quantum magnetism at the frontier of classical simulations, arXiv (2025). DOI: 10.48550/arxiv.2503.20870