In the same way that life on Earth transitioned from aquatic organisms to land-dwelling creatures, soft robotics is experiencing a similar evolution. Recent research at Cornell University has made significant strides in developing soft robots that integrate battery systems directly into their structures. This approach, known as embodied energy, is transforming soft robots, making them lighter, more efficient, and cost-effective. By drawing inspiration from living creatures like worms and jellyfish, these robots demonstrate a seamless blend of biology and engineering, bringing the next generation of soft robotics closer to reality.
A Modular Worm Robot: Bringing Soft Robotics to Land
One of the most innovative prototypes to come out of this research is a modular worm robot developed by Cornell’s Organic Robotics Lab. This robot is a direct descendant of earlier aquatic soft robots, designed to mimic creatures like the lionfish and powered by hydraulic systems, which use a circulating fluid to store and transfer energy. The primary innovation in the worm robot is its modular structure, which consists of interconnected segments or “pods” that each contain a motor and tendon actuators. These actuators allow the worm to compress, expand, and move along the ground in a manner similar to natural worms.
Unlike its aquatic predecessors, which were buoyant and didn’t require a rigid skeleton for support, the land-based worm robot had to adapt to the gravity and friction of terrestrial environments. As a result, its design incorporates embodied energy — the energy source and actuators are integrated within its body, making it more efficient and reducing its weight. This advancement allows for longer travel distances and greater flexibility in movement, with applications ranging from exploration to repairs in narrow, hard-to-reach spaces, such as pipes or tunnels.
The Jellyfish Robot: A Leap in Fluid-Driven Movement
Another standout robot from this research is the jellyfish robot, a collaboration between the Organic Robotics Lab and the Archer Group. This robot, designed to mimic the undulating movement of a jellyfish, features an innovative redox flow battery (RFB) as its core power source. The RFB is responsible for providing energy to the robot’s tendon, which changes the shape of the jellyfish’s bell, causing it to contract and expand. This motion propels the jellyfish robot upward, allowing it to navigate through water with ease.
What sets the jellyfish robot apart is its advanced battery design. The research team led by Lynden Archer, the James A. Friend Family Distinguished Professor of Engineering at Cornell, developed a dual redox flow battery system, combining zinc iodide (ZnI₂) and zinc bromide (ZnBr₂). These batteries are unique because they help minimize the buildup of dendrites—tiny crystal formations that can interfere with the performance of conventional batteries. By using graphene as a coating, the researchers ensured that the zinc ions were more evenly distributed during charge and discharge cycles, significantly improving the efficiency and power density of the battery.
Additionally, the incorporation of bromine in the ZnBr₂ battery helps improve ion transport, further enhancing performance. With these modifications, the jellyfish robot can operate for around 90 minutes, longer than previous versions, and with greater speed and agility. The jellyfish robot’s lightweight, fluid-driven system makes it ideal for applications in ocean exploration, where it can travel with the current, dive, and ascend to the surface to communicate, creating a cost-effective solution for gathering data from underwater environments.
Redox Flow Batteries: The Heart of Soft Robot Power
At the core of both the worm and jellyfish robots is the redox flow battery, which functions as the “heart” of these soft machines. Redox flow batteries operate by using electrolytic fluids that undergo a chemical reaction known as reduction and oxidation (redox). In simple terms, these reactions involve the transfer of electrons between materials, releasing and storing energy in the process.
For the jellyfish robot, the energy released by the RFB powers the tendon, which, when pulled, alters the shape of the robot’s bell, allowing it to move. The flow of electricity generated by the redox reaction drives the robot’s motion, giving it the agility to navigate and travel. In the case of the worm robot, the redox flow batteries provide both the energy needed for movement and the hydraulic force to drive the worm’s actions, making them an essential part of the robot’s overall function.
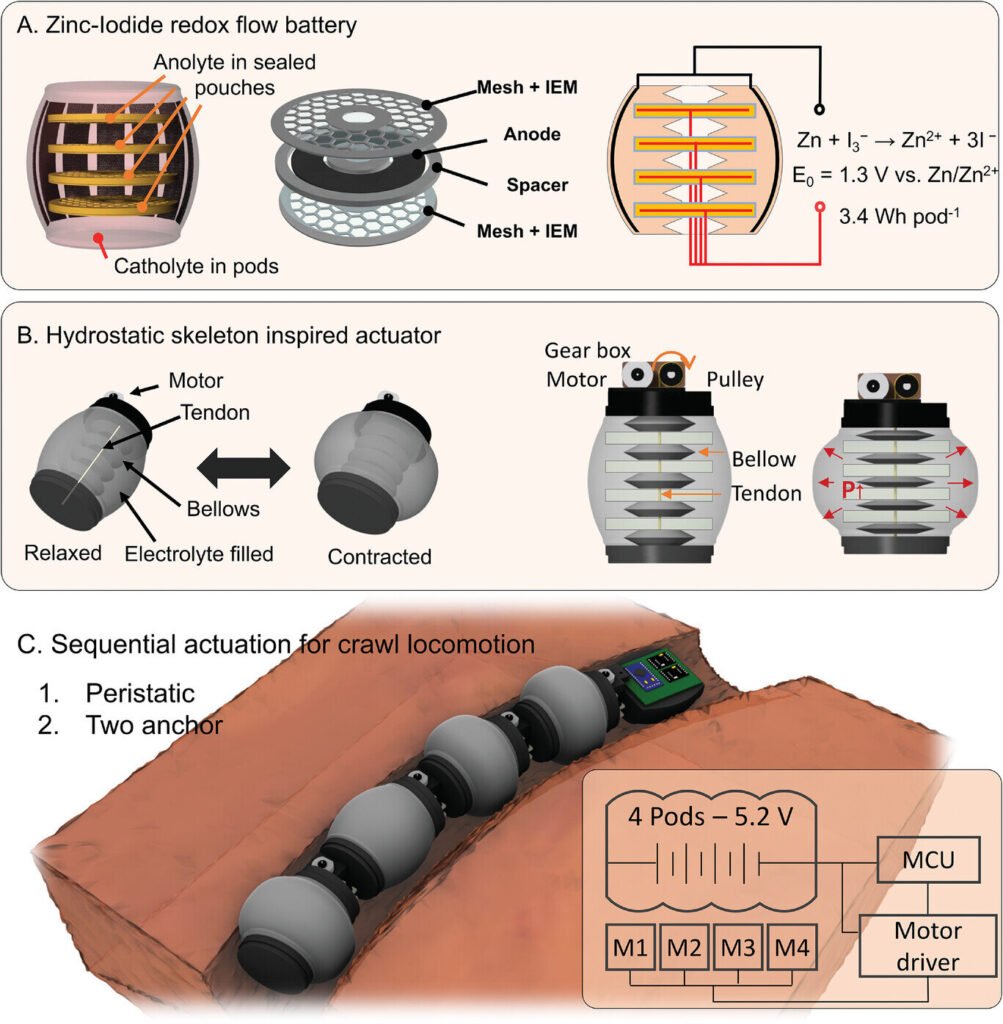
The Modular Worm Robot: A New Mode of Movement
The worm robot represents a major leap in soft robotics, primarily due to its modular design. Each of the robot’s segments, or pods, contains an individual motor and actuator, which are responsible for moving the robot forward. The robot’s movement is achieved through hydraulic fluid, which both powers the system and provides the force required for locomotion.
The worm robot has two primary movement modes: ground-based crawling and vertical climbing. In crawling mode, the robot contracts its pods in sequence to inch forward. In vertical climbing mode, it utilizes a technique known as two-anchor crawling, where the worm anchors itself at two points and moves by alternately shifting its position. This flexibility in movement makes the worm robot ideal for navigating long, narrow passageways like pipes or cables. The worm is not particularly fast — it would take about 35 hours to cover 105 meters on a single charge — but it is more efficient than other similar robots and can cover greater distances due to its low weight and embedded energy system.
Practical Applications for Soft Robotics
Both the worm and jellyfish robots demonstrate significant potential for real-world applications. For the worm robot, the primary application is in environments that are difficult for humans or traditional machines to access. Pipe inspections, repair operations, and even search-and-rescue missions in confined spaces could all benefit from the worm’s unique ability to move through tight, winding areas. Its embodied energy system also ensures that it can travel for long periods without needing frequent recharging, making it an invaluable tool in scenarios where battery life and portability are crucial.
The jellyfish robot, on the other hand, has applications in ocean exploration, where it can float with the current and travel long distances without the need for external propulsion. Its fluid-driven system makes it highly energy-efficient, and it can travel without a fixed power source, making it ideal for collecting environmental data or even underwater communication.
Future Prospects and Evolution of Soft Robots
Looking ahead, Rob Shepherd, professor of mechanical and aerospace engineering and the lead researcher behind both projects, anticipates that embodied energy robots will continue to evolve. One potential future development is the integration of lithium-polymer batteries with the existing fluid-driven technology, which would allow the robots to gain even greater power capacity and energy efficiency. Moreover, Shepherd envisions robots that not only crawl or swim, but also have skeletons and can walk, opening up even more possibilities for these machines in practical applications.
“We’re moving toward machines that are more like us,” said Shepherd, “imperfect organisms with a lot of flexibility, but still performing really well.”
Contributions and Collaborations
The worm and jellyfish robot projects were collaborative efforts between several prominent researchers, including Lynden Archer, the James A. Friend Family Distinguished Professor of Engineering and dean of Cornell Engineering, and Chong-Chan Kim, the lead author of the worm robot study. The jellyfish study, published in Science Advances in November 2023, was led by Xu Liu, a Ph.D. student at Cornell, and included contributions from various researchers across Cornell Engineering and Technion-Israel Institute of Technology.
The worm robot study was published in Advanced Materials, with Anunth Ramaswami, a student at Cornell, co-authoring the research. These groundbreaking advancements in soft robotics highlight the continued progress of embodied energy systems, pushing the boundaries of what robots can achieve and how they can function in both natural environments and human-engineered systems.
Conclusion
As soft robotics continue to evolve, the developments at Cornell represent a new frontier in robot design and energy systems. By integrating power sources directly into the structure of the robots, the team has created lightweight, efficient, and long-lasting robots that can move fluidly and adapt to different environments. With applications in ocean exploration, search-and-rescue operations, and even future space exploration, the potential for these robots is vast. The worm and jellyfish robots are just the beginning of an exciting new era in robotics, where biological inspiration and engineering innovation converge.
References: Chong‐Chan Kim et al, Soft, Modular Power for Composing Robots with Embodied Energy, Advanced Materials (2025). DOI: 10.1002/adma.202414872
Xu Liu et al, The multifunctional use of an aqueous battery for a high capacity jellyfish robot, Science Advances (2024). DOI: 10.1126/sciadv.adq7430