Quantum systems are strange beasts. Unlike the predictable machines of classical physics, they behave more like slippery phantoms—simultaneously existing in multiple states, vanishing into uncertainty the moment you try to pin them down. But their power lies precisely in this strangeness. Superposition, entanglement, and coherence form the foundation of quantum mechanics—and the basis for revolutionary technologies like quantum computing and quantum communication.
However, these magical properties are fragile. The moment a quantum system interacts with its environment, it begins to lose coherence in a process known as decoherence—and with it, its uniquely quantum features fade away. A major culprit behind decoherence is dissipation: the irreversible loss of energy from a system. Traditionally, dissipation has been viewed as the ultimate adversary of quantum behavior.
But what if we’ve been looking at dissipation the wrong way?
In a groundbreaking study published in Nature Physics, a team of researchers at Tsinghua University flipped the narrative. Instead of treating dissipation as the villain in the quantum story, they explored how it could be used as a scientific lens—a tool to peer into the deep and mysterious world of strongly correlated quantum matter.
Quantum Leverage: Using Loss to Reveal Hidden Order
“We were inspired by the growing interest in open quantum systems and non-Hermitian physics,” explained Yajuan Zhao, the study’s first author. “These areas suggest that dissipation isn’t always the enemy—it can actually uncover properties of quantum systems that are otherwise hard to measure.”
This unconventional thinking led Zhao and her team to devise a novel approach. They aimed to detect intrinsic many-body correlations—the complex interrelationships between particles in systems where traditional theories break down. Their focus was on one-dimensional (1D) quantum gases, a platform where quantum interactions are so strong that individual particles no longer behave independently.
Normally, physicists rely on Hermitian operators—a staple of textbook quantum mechanics—to analyze systems. But these tools fall short in open systems where energy is not conserved. The Tsinghua team boldly ventured beyond this framework, using dissipative dynamics as their guide.
The Experiment: Lighting Up the Quantum Shadows
To test their hypothesis, the researchers cooled Rubidium-87 (Rb-87) atoms to near absolute zero, forming ultracold 1D Bose gases. These atoms were trapped in thin tubes, like strands of spaghetti, created by a 2D optical lattice—essentially a crisscrossing web of laser beams that confined the atoms into a 1D world.
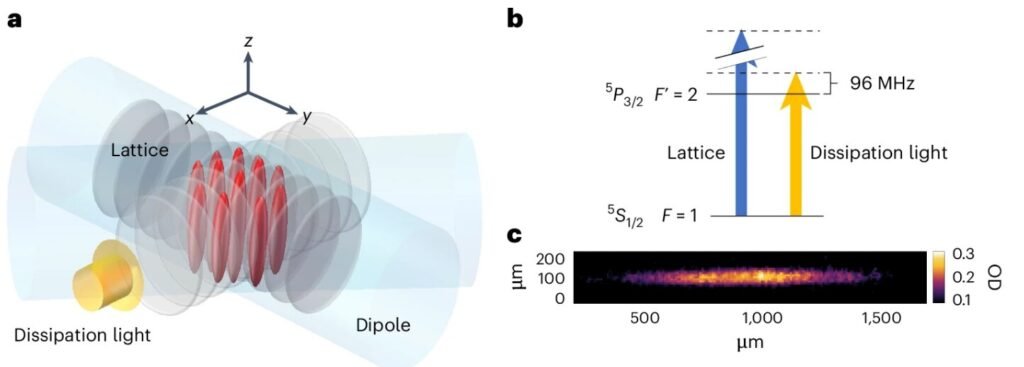
Then came the twist: instead of protecting the system from energy loss, they deliberately introduced dissipation using a carefully tuned near-resonant laser beam. This light caused a controlled one-body loss, where atoms were kicked out of the system one at a time. By tracking the number of atoms over time using absorption imaging, they observed how the quantum gas “dissolved” under this engineered leak.
And here’s where it gets fascinating: the atom number didn’t simply decay in the predictable exponential fashion seen in most dissipative systems. Instead, they observed a stretched-exponential decay—a clear signature that something deeper was at play.
The Universal Signature of Correlation
This decay wasn’t random. It followed a universal law, dictated not by the characteristics of the laser or the temperature of the gas, but by the dimensionless interaction strength between particles in the 1D system. The key parameter? The stretched exponent, which the researchers showed was connected to a critical theoretical quantity: the anomalous dimension of the Luttinger liquid.
Luttinger liquid theory is a framework used to describe the strange collective behaviors in 1D quantum systems, where the usual rules of particle physics no longer apply. Instead of thinking of particles like billiard balls, Luttinger liquids treat them as waves—deeply interlinked and inseparable.
What Zhao and her colleagues found is that the stretched exponent provides a direct experimental window into these exotic properties, particularly the spectral function—a notoriously elusive quantity that reveals how particles interact and respond to energy inputs. Measuring this in closed systems is extremely difficult, but dissipation provided a shortcut.
Rewriting the Rules of Quantum Measurement
“This is what makes our result so exciting,” Zhao noted. “We’ve shown that dissipation isn’t just a nuisance—it’s a resource. By using controlled energy loss, we can probe internal correlations that are almost impossible to access otherwise.”
Their approach challenges long-standing assumptions in quantum physics. Traditionally, measurements are made under linear response theory, which assumes that a system’s response to an external probe is proportional to the strength of that probe—an idea that holds in closed, isolated systems. But this doesn’t hold true when dealing with open quantum systems—those continuously exchanging energy and information with their environment.
Zhao’s experiment offers a new paradigm: using dissipative probes to extend quantum measurement techniques to non-Hermitian, open systems. This not only enriches our understanding of quantum many-body physics but could also spark entirely new classes of experiments.
Implications for the Future: Quantum Materials and Beyond
What makes this study even more exciting is its potential impact beyond ultracold gases.
The behavior of strongly correlated quantum matter—from high-temperature superconductors to quantum spin liquids—is one of the most important and least understood frontiers in physics. These materials exhibit exotic properties like resistance-free current flow and long-range entanglement, but their inner workings remain mysterious. Probing them using conventional tools has often hit a wall.
But with the method pioneered by the Tsinghua team, researchers now have a new handle for studying these elusive systems. Dissipative techniques could help investigate phenomena like spin-charge separation, where an electron splits into independent components of spin and charge, or even the strange behaviors seen in non-Fermi liquids, which defy traditional models of electron behavior.
Zhao and her colleagues are already looking ahead: “Our next goal is to use this method to study these more complex quantum systems. We believe dissipation can help us answer some of the biggest open questions in modern condensed matter physics.”
Turning Loss into Light: A Quantum Philosophy Shift
In a broader sense, this work represents a philosophical shift in how scientists think about quantum systems. Instead of fighting dissipation as an unavoidable evil, researchers are learning to dance with it, turning what was once seen as noise into a signal.
It’s a reminder that in quantum physics, paradox is often the rule—not the exception. And sometimes, what appears to be destruction can actually illuminate hidden order.
As we build the future of quantum technologies, from computing and sensing to communication and materials design, embracing this duality—of loss and revelation, decay and insight—may be the key to unlocking new worlds.
Reference: Yajuan Zhao et al, Universal dissipative dynamics in strongly correlated quantum gases, Nature Physics (2025). DOI: 10.1038/s41567-025-02800-4.