In the infinite stage of the cosmos, stars dominate the spotlight. They shine so brightly that their lesser-known companions—planets, potentially teeming with alien life—vanish into their radiance. For decades, this stellar glare has posed one of the most formidable barriers to one of modern astronomy’s most tantalizing quests: directly imaging Earth-like exoplanets orbiting distant stars. Now, researchers from the University of Arizona may have cracked a piece of that cosmic puzzle with a groundbreaking new optical device that dances along the very edge of quantum physics.
Welcome to the era of the next-generation coronagraph—a precision optical instrument that could allow us to see what was once thought invisible.
The Challenge of Stellar Blinding
Imagine trying to spot a firefly buzzing next to a lighthouse from a thousand miles away. That’s the kind of contrast astronomers face when attempting to detect Earth-sized exoplanets orbiting Sun-like stars. The planet is often up to a billion times dimmer than the star it orbits, especially if it lies within the “Goldilocks zone,” the region where liquid water—and potentially life—might exist.
“The brightness of the star essentially drowns out the signal from the exoplanet,” explains Nico Deshler, lead researcher and optical physicist at the University of Arizona. “It’s not just a matter of focusing the telescope better—it’s about rethinking how we see.”
And rethink they did.
A New Kind of Optical Sorcery
Published in the journal Optica, the team’s innovation lies in an entirely new coronagraphic design—one that leverages the exotic behavior of light at the quantum level to separate the faint whisper of a planet from the deafening shout of its star. Their system, unlike traditional coronagraphs, doesn’t just block starlight—it siphons it away, selectively and precisely, before the image is even formed.
At the heart of this innovation lies a piece of optical wizardry known as the spatial mode sorter.
Light, though commonly understood in terms of color or wavelength, also carries another less intuitive characteristic: spatial mode. Just as a piano note resonates with a particular frequency and pattern, light emitted from different positions in space (such as a star versus a nearby planet) travels in unique spatial configurations.
Using this principle, the researchers engineered a setup that can sort the incoming light by these patterns. The starlight—on-axis and intense—follows one predictable spatial mode. The planet light, arriving from a slightly offset angle, follows another. By directing the star’s mode into a trapdoor and letting the planet’s light pass through undisturbed, the coronagraph acts like a cosmic bouncer—ushering in the dim guests and keeping the loud ones out.
This approach doesn’t just remove the starlight—it reconstructs the planetary signal afterward using an inverse mode sorter, effectively piecing together the planet’s image from the filtered data. The result is not just a detection of light intensity, but a full image, rich with spatial information.
Smashing Through the Resolution Barrier
One of the most extraordinary aspects of the device is its ability to defy the classical resolution limits of the telescope. Normally, the angular resolution—the ability to distinguish two closely spaced objects—is governed by the telescope’s aperture and the wavelength of light, known as the diffraction limit. But by using optical preprocessing techniques, such as spatial mode sorting, the researchers found a way to cheat the system.
“In theory, we’ve shown that this coronagraph can resolve objects up to 50 times closer than what the telescope’s resolution limit would normally allow,” said Deshler.
In practical terms, this means being able to image exoplanets in tight, short orbits—exactly the kinds of planets that are hardest to see but most intriguing for the search for life.
A Lab-Built Solar System
To test their creation, the team built a miniature mock solar system in their lab—an artificial star and an exoplanet with a simulated contrast ratio of 1000:1. They adjusted the artificial planet’s position to simulate an orbit, allowing it to pass in front of the star in a series of frames, much like a planet might transit its host star in real space.
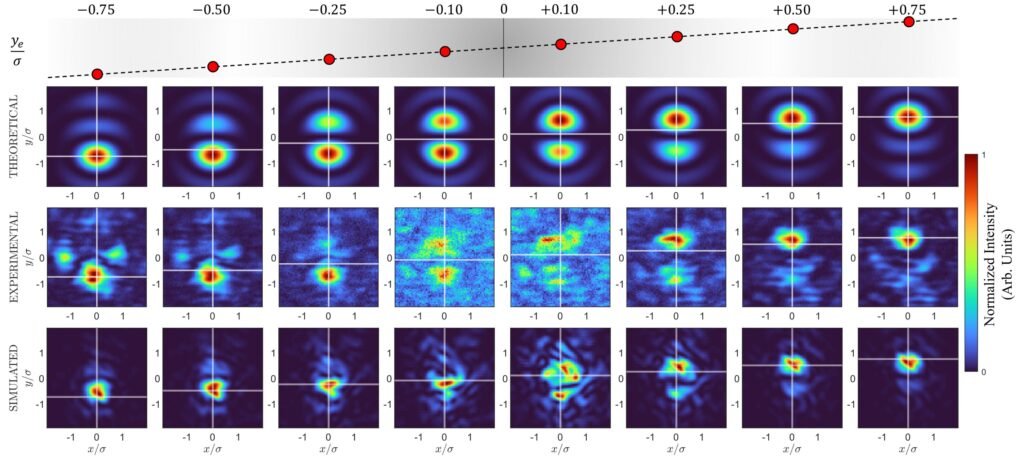
Traditional imaging techniques would have failed to pick up the faint planet against such overwhelming brightness. But using their new coronagraph, the researchers successfully captured frames that allowed them to pinpoint the planet’s position—even when it was closer than the resolution limit would typically permit.
This sub-diffraction tracking opens the door to dynamic exoplanet imaging—not just static snapshots, but evolving orbital paths, atmospheric analyses, and even long-term habitability studies.
Battling Crosstalk and Optical Interference
Of course, no frontier technology comes without hurdles. One of the main challenges the team encountered was crosstalk, a type of optical interference where light meant for one mode bleeds into another, muddying the clarity of the final image. In the delicate business of exoplanet detection—where contrast ratios can range into the millions or billions—such contamination can be devastating.
To address this, the researchers are now focused on improving the fidelity of the spatial mode sorter, refining its ability to cleanly distinguish and separate light streams. Even small reductions in crosstalk can yield exponential improvements in image clarity and detection probability, especially for faint planets orbiting closely around massive, blinding stars.
From Starlight to Signatures of Life
One of the most exciting implications of this technology is its potential to detect biosignatures—the chemical fingerprints of life. When astronomers image an exoplanet, they don’t just want to see it; they want to analyze its atmosphere, hunt for water vapor, oxygen, methane, and other telltale signs of biological activity.
“Images provide context,” Deshler says. “They help us understand an exoplanet’s orbit, its relationship to the star, and whether there are other light-scattering objects like exozodiacal dust in the system.”
A full image, as opposed to a mere detection, is the golden ticket. It means we can study cloud cover, track weather patterns, and perhaps, one day, glimpse the glow of alien cities—if such civilizations exist.
Preparing for the Habitable Worlds Observatory
This coronagraphic breakthrough comes at a pivotal time. NASA’s upcoming Habitable Worlds Observatory (HWO) is being designed specifically to hunt for exoplanets in the habitable zones of nearby stars. Coronagraph designs are at the center of the mission, and the competition to develop the most effective light-blocking strategies is fierce.
Deshler’s team believes their method could complement or even enhance the HWO’s capabilities. By bypassing traditional resolution limits and maximizing the signal from off-axis sources, their coronagraph could greatly expand the list of candidate planets the observatory might image.
Beyond Astronomy: A Quantum Future
Interestingly, the implications of this technology stretch well beyond space science. The same mode-sorting techniques used to isolate planetary signals can also be applied in other high-resolution imaging fields, such as quantum sensing, biomedical imaging, and secure optical communications.
Imagine a future where your doctor can peer into your body with the same clarity astronomers use to peer into alien solar systems—or where quantum data is transmitted through networks filtered with light-mode sorters that eliminate interference and noise.
By mastering the spatial behavior of light, we’re not just looking deeper into space—we’re looking deeper into everything.
The Dawn of Direct Discovery
For centuries, the stars have overshadowed their planets, hiding them in brilliance and distance. But with this new coronagraph, astronomers are poised to lift the veil on these hidden worlds, bringing us closer than ever to answering one of humanity’s oldest questions: Are we alone?
Deshler and his team have offered a powerful new tool—not just to astronomers, but to all seekers of hidden truths. It’s a reminder that light, though sometimes blinding, can also be bent, sorted, and sculpted to reveal secrets beyond imagination.
As our telescopes evolve, so too does our vision of the cosmos. And with every photon captured, every speck of dim light revealed, we move one step closer to the exoplanets—and perhaps the neighbors—we’ve been searching for all along.
Reference: Nico Deshler et al, Experimental demonstration of a quantum-optimal coronagraph using spatial mode sorters, Optica (2025). DOI: 10.1364/OPTICA.545414